60 Respiratory Structures and Functions
Gross Anatomy of the Upper Respiratory Tract
Nose
The external portion of the nose begins at the base of the frontal bone and extends over the maxilla, with the nasal bone providing the bridge of the nose. Extending from the nasal bone is a collection of hyaline cartilages that make up the bulk of the nose. The medial region of the nose consists of a central septal cartilage with two lateral processes. The tip of the nose contains the major alar cartilage. Two minor alar cartilages are found at the sides and base of the lateral septal cartilages. Dense fibrous connective tissue is found under the skin of the sides lateral aspects the nose, away from the cartilage. Variations in the size of a person’s nose, or its form, are due to differences in the various cartilages.
The visible nose is actually the entryway into the nasal cavity, where the major functions of the nose occur. The openings to the nose, the nares, are lined with coarse hairs to aid in filtration of particulate matter. The area immediately inside the nares, the vestibule, contains a large number of sebaceous glands, sweat glands, and hair follicles.
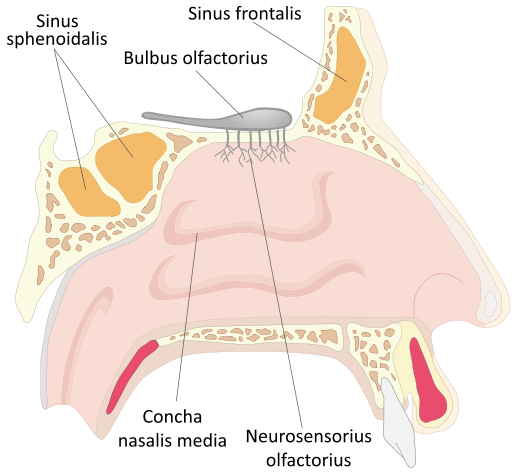
The nasal cavity is divided into right and left sides by the nasal septum. This dividing wall’s anterior portion is made of cartilage; bone contributed by the vomer and part of the ethmoid bones of the skull make up the posterior. The roof of the nasal cavity consists of parts of the ethmoid and sphenoid bones. Its floor, the palate, forms the roof of the mouth. It is separated into the hard and soft palate. The anterior hard palate is formed from the maxillary process of the palatine bone. The posterior soft palate does not contain bone and moves during swallowing to close off the nasal cavity to prevent material from entering it from the mouth.
Extending from the nasal septum are three pairs of C-shaped structures called conchae. The superior, middle, and inferior conchae extend the length of the nasal cavity. They are covered by a mucus membrane that contains a large number of mucus-secreting cells and blood vessels. A bloody nose highlights the richness of the blood supply to the membranes on the conchae. The conchae serve as baffles to increase the surface area of the nasal cavity. The mucus glands and blood vessels aid in humidifying and warming the air coming into the body.
There are two types of epithelial coverings in the nasal cavity: respiratory epithelium and olfactory epithelium. Both types of epithelia appear very similar when viewed through a light microscope. The olfactory mucosa found in the roof of the cavity detects odors. This epithelial layer contains specialized nerve cells that detect odors and transmit impulses to the first cranial nerve. The respiratory epithelium that covers the rest of the nasal cavity is also found through most of the respiratory tract and is pseudostratified, ciliated, columnar epithelia.
Paranasal Sinuses
Within the bones surrounding the nasal cavity are paranasal sinuses (a sinus is a hollow area), which function to make the skull lighter as well as moisten and warm incoming air. These sinuses frequently become filled with excess fluid when a person has a head cold. Since the paranasal sinuses serve as resonators for speech and sound it is not surprising that the sound of the voice becomes altered when they are filled with fluid or swollen. The frontal, sphenoid, ethmoid and maxillary bones all contain sinus cavities.
Pharynx
As the air passes posteriorly through the nasal cavity, it enters the pharynx. This structure encompasses three distinct areas and connects the nasal passage to the larynx in the throat. It extends about 13 centimeters or 5 inches from the base of the skull to the level of the sixth cervical vertebrae. The wall of all three portions contains two layers of skeletal muscle. The inner layer is arranged in a circular pattern, and the outer layer is arranged longitudinally.
Nasopharynx
The superior section of the pharynx is called the nasopharynx. It is posterior to the nasal cavity and inferior to the sphenoid bone. This chamber shares the same epithelia as the nasal cavity and acts only as a conduit for air. The other two portions of the pharynx contain shared passages with the digestive tract and act as conduits for both air and food. The nasopharynx closes off during swallowing by raising the soft palate. Also found in this area are structures associated with immune system. The paired pharyngeal tonsils, also known as the adenoids, lie in the posterior wall of the nasopharynx.
Oropharynx
The second portion of the pharynx is the oropharynx. It runs from the soft palate to the epiglottis and is posterior to the oral cavity. The opening from the oral cavity to the oropharynx is called the oropharyngeal isthmus (Isthmus of Fauces). A difference between the nasopharynx and oropharynx is the epithelial covering of the passages. In comparison to the nasopharynx, which is lined with columnar epithelia, the oropharynx is covered by stratified squamous epithelia, that are often found covering areas which are subject to a great deal of frictional wear. The many layers of epithelial cells serve to protect the underlying tissues from being damaged by the food and material coming into the passage from the mouth.
Laryngopharynx
The third portion of the pharynx is the laryngopharynx. The shortest of the three parts of the pharynx, it runs inferiorly from epiglottis and ends superior to the esophagus. The laryngopharynx carries both air and food and is lined with stratified sqaumous epithelia.
Tonsils
The paired pharyngeal tonsils, also known as the adenoids, lie in the posterior wall of the nasopharynx. The other tonsils, the tubal tonsils, protect the auditory tubes and middle ear against entering microorganism. They are located at the opening of the tube that connects the middle ear to the nasopharynx. The auditory or Eustachian tube equilibrates air pressures between the environment and the middle ear. The orientation of the auditory tube changes during infancy and early childhood. The tube is oriented horizontally until around age two. Consequently, it frequently serves as a point of entry for bacteria in children less than two years of age. Around age two, the auditory tube changes course and slants down from the ear, allowing fluid to drain more readily. Without the fluid to act as a growth medium and conduit to the middle ear, older children usually experience few ear infections.
The paired palatine tonsils lie along the two sides of the Isthmus of Fauces in the oropharynx. The lingual (lingual meaning tongue) tonsils are not in the oropharynx, but are located at the base of the tongue. Collectively, the tonsils aid in filtering foreign material that could do harm.
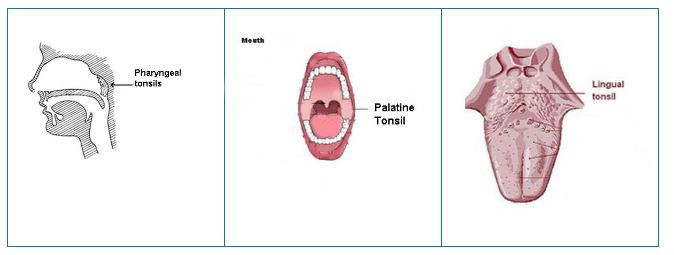
Larynx
After air leaves the pharynx, it enters the larynx, a complex structure that extends from the laryngopharynx and the hyoid bone to the trachea. It is about 5 cm (2 in.) long. In addition to providing a passageway for air, the larynx directs air and food to their appropriate tubes. The airway is blocked by closing off the opening of the trachea with the epiglottis upon swallowing. The vocal cords, which are used in making sound and speech, can be found within the larynx. The epithelial lining of the larynx exhibits two different arrangements. Initially stratified squamous epithelia lines from the laryngopharynx to the vocal cords. Inferior to the vocal cords the epithelial lining shifts to pseudostratified, ciliated, columnar epithelia. The nine cartilage structures found in the larynx provide key anatomical landmarks. They function to maintain an open airway. Eight of the cartilages are composed of hyaline cartilage.
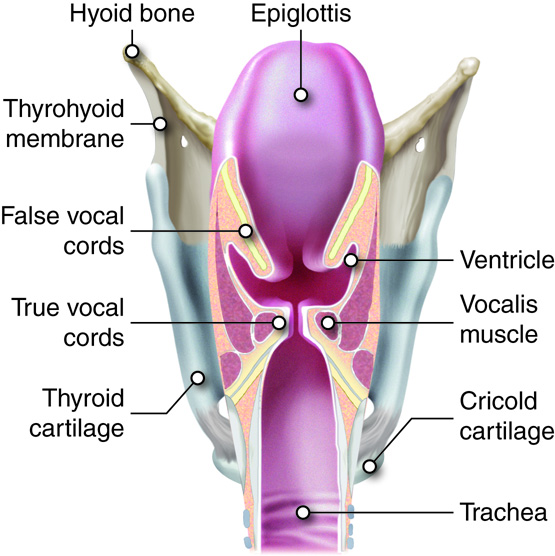
Epiglottis
The epiglottis is made of elastic cartilage and covered with stratified squamous epithelia. It connects loosely to the tongue, the hyoid bone, and the rim of the thyroid cartilage. The epiglottis is normally open, allowing air to freely flow into the rest of the larynx and the trachea. When a person swallows, the front of the epiglottis is raised, and the posterior portion descends, covering the glottis, which is the opening to the vocal cords and trachea. This movement directs food and water to the esophagus and prevents it from entering the bottom portion of the larynx and the upper trachea. If someone tries to talk or laugh and swallow at the same time, the air used to talk or laugh forces the epiglottis open, the swallowed material “goes down the wrong tube,” and choking results.
Thyroid cartilage
The thyroid cartilage is the largest of the cartilages of the larynx and is found at the front of the larynx. In the fetus the cartilage originates as two separate plates that fuse before birth. This roughly triangularly shaped cartilage contains the laryngeal prominence, commonly known as the “Adam’s apple”. The laryngeal prominence becomes more prominent in males during puberty as the larynx widens and the voice deepens. The thyroid cartilage connects to the hyoid bone by the thyrohyoid membrane or ligament.
Cricoid cartilage
Inferior to the thyroid cartilage is the cricoid cartilage. It connects superiorly to the thyroid cartilage by the cricothyroid ligament and inferiorly to the trachea by the cricotracheal ligament. When an occlusion of the upper respiratory tract occurs and a tracheostomy is performed to facilitate breathing, the cricothyroid ligament must be punctured.
Arytenoids cartilages, cuneiform cartilages, corniculate cartilages
The next six cartilages are found in three pairs. The first pair, the arytenoids cartilages, anchor the true vocal cords. The second pair are the cuneiform cartilages, and the third pair are the corniculate cartilages. All three pairs of cartilages are found in the lateral and posterior walls of the larynx. Except for the epiglottis, the arrangement of the cartilages of the larynx ensure that the passages through the larynx remain open.
Vocal cords
Two pairs of folded tissue can be observed in the larynx immediately inferior to the epiglottis. These are the false and true vocal cords respectively. The false vocal cords do not function in making sounds or speech but aid in closing the glottis, which opens to the rest of the larynx and the respiratory system. Below the false vocal cords are the true vocal cords. The true cords run from the arytenoids to the thyroid cartilage. They are reinforced with elastic fibers and vibrate when adequate air is forced through the gap between them, resulting in sound. Pitch control is achieved by adjusting the tension on the cords. Lessening the tension lowers the pitch. During puberty in males the cords are usually lengthened making for a deeper sound. Actual speech is achieved with the coordination of muscles in the pharynx, face, tongue, soft palate, and lips.
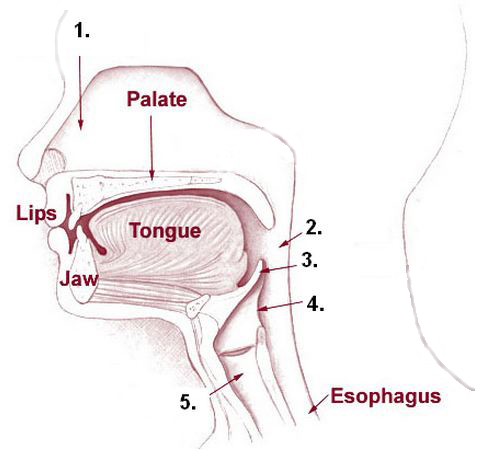
Gross Anatomy of the Lower Respiratory Tract
Trachea
The flow of air continues from the larynx to the trachea or windpipe. The trachea is about 10 cm (4 in.) long and 2 cm (3/4 in.) wide and extends from the larynx to the level of the fifth thoracic vertebrae. The presence of 16 to 20 C-shaped pieces of hyaline cartilage located along the trachea prevent this airway from closing. The last cartilage in the trachea exhibits a projection from the anterior surface that extends into the lumen. This projection, the carina, is very sensitive to particulate material and causes coughing when stimulated. The openings of the C-shaped cartilages face the posterior of the trachea and contain the trachealis smooth muscle. This muscle constricts when someone coughs, increasing the force of the cough. The trachealis muscle also constricts during an asthmatic reaction, shrinking the airway and making it harder to breathe.
Structurally the trachea consists of three concentric tissue layers: the mucosa, submucosa, and adventitia. This arrangement is continuous throughout the remainder of the upper respiratory system. The layer facing the lumen, the mucosa, is composed of pseudostratified ciliated columnar epitheliia. The submucosa contains blood vessels, nerves, assorted connective tissues, and mucus glands. The outer adventitia is mostly areolar connective tissue that is continuous with the hyaline cartilage and connects the trachea to the surrounding structures and tissues.
The Bronchi and Bronchioles
The bronchial tree is so named because it resembles a tree that has been turned upside-down. The trachea would be the trunk, the progressively smaller bronchi and bronchioles are the branches, and the alveoli are the leaves. Branching of the trachea into the right and left primary bronchi occurs after the last cartilage in the trachea at the level of the seventh thoracic vertebrae. The right primary bronchus is wider, shorter, and more vertical than the left primary bronchus because the left primary bronchus and lung must accommodate the heart. The primary bronchi branch to form the secondary or lobar bronchi. There are three secondary bronchi on the right and two on the left. The secondary bronchi continue to branch into the tertiary or segmented bronchi, which also continue the branching pattern. Approximately 23 successive branches lead to the bronchioles. A bronchiole is a tube with a diameter of less than 1 millimeter (mm). When the measurement reaches less than 0.5 mm, a bronchiole is termed a terminal bronchiole.
The bronchial tree is lined by pseudostratified, ciliated, columnar epithelia with goblet cells dispersed among the columnar cells. Plates of cartilage are found in the walls of the bronchial tree, with the amount of cartilage and the number of plates decreasing as the bronchi and bronchioles become smaller. The terminal bronchioles do not contain cartilage plates, these tubes are small enough to stay open without cartilage. Smooth muscle is found throughout the system, even into the respiratory zone.
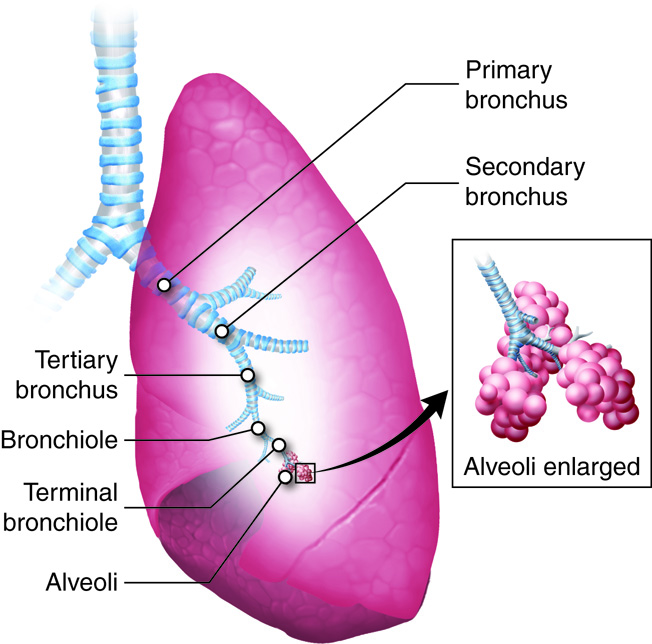
The Lungs
The lungs are two of the largest organs of the body, but they are among the lightest. Along with the heart, the lungs take up nearly all of the space in the thorax, superior to the diaphragm. As an organ, the lung is made up of airway tubes and alveoli, giving it little weight. Elastic connective tissues in the stroma of the lungs allow them to expand with incoming air and recoil when expelling air. The lungs contain a large amount of surface area in order to efficiently support the exchange of oxygen and carbon dioxide.
The hilus (meaning depression or pit) of the lungs is an indentation on the medial side of the lungs and the point of entry of blood vessels, primary bronchi, nerves, and lymphatics. This collection of vessels and nerves makes up the root of the lung. The tip or apex of the lungs is a blunted point found just above the clavicles. The posterior, lateral, and anterior sides of the lungs are surrounded by the ribs. These areas are called the costal surfaces of the lungs referring to the costal cartilage surrounding them. The flat, inferior surface of the lungs is found superior to the diaphragm and referred to as the base of the lung. Since, the liver is found on the right side of the body and inferior to the diaphragm, the insertion of the diaphragm is slightly raised on the right. Consequently the right lung is usually slightly shorter than the left. The lungs extend from the first costal cartilage to the tenth thoracic vertebrae.
The lungs consist of a right lung and a left lung. Even though the right lung is slightly shorter than the left, the left lung has about 10 percent less mass than the right due to the cardiac notch on the medial side of the left lung. The heart is tucked into this notch. The heart, the right lung, and the left lung, are each located in their own anatomical compartment in the upper thorax. The right lung is divided into three lobes. A horizontal fissure separates the superior and middle lobes, and an oblique fissure separates the middle and inferior lobes. The smaller left lung contains only two lobes. An oblique fissure separates the superior and inferior lobes on this side.
Each lobe is divided into bronchopulmonary segments separated by connective tissue septa. There are a total of 10 of these segments and each contains a tertiary bronchiole, a pulmonary and bronchial artery, and a lymphatic branch. The presence of these segments aids in further isolating parts of the lungs to prevent the spread of infection or disease. Connective tissue further divides the segments into lung lobules, the smallest anatomical unit in the lungs. A lobule is hexagonal in shape and less than a centimeter in diameter. Each lobule contains a terminal bronchiole and its associated alveoli. The connective tissue associated with lobules may be blackened by tobacco smoke or pollution from the environment.
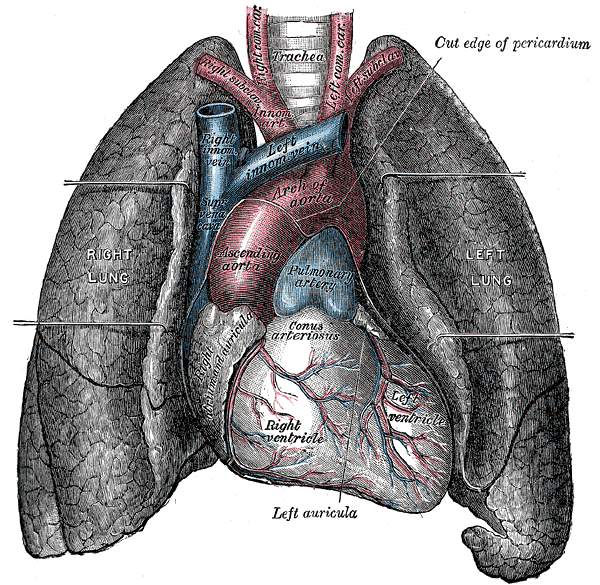
The image below compares the lungs from a smoker with those from a non-smoker.
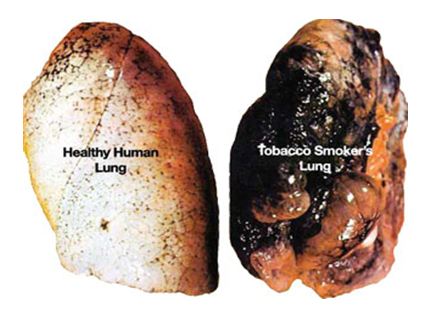
Usually, lungs are a pink with a relatively smooth surface. The lungs on the right are blackened and irregularly shaped from the accumulation of material inhaled with tobacco smoke. This material can be cleared from the lungs, but only over a long period of time and only if the person stops smoking. Cigarette smoking causes the deaths of over 440,000 people in the United States each year, including people exposed to second-hand smoke. The financial losses associated with these deaths are in excess of 200 billion dollars a year.
There are over 4,000 chemical compounds that are created when the more than 600 chemical ingredients in cigarettes are burned and inhaled. More than 50 of the chemicals produced by the burning of a cigarette are classified as carcinogens. A partial list of these chemicals includes: acetone, acetic acid, ammonia, arsenic, benzene, butane, cadmium, carbon monoxide, formaldehyde, lead, naphthalene, methanol, nicotine, tar, and toluene.
The direct effect of many of the chemicals in the smoke is to paralyze and destroy cilia. When the cilia cannot function, mucus accumulates in the lung tubules. Opportunistic bacterial and other microorganisms utilize the mucus to grow and colonize the lungs. Many of these organisms create pathologic conditions inside the lung.
Smoker’s cough is a condition that exists because the mucus and other debris accumulate in the lung tissue. These accumulations stimulate the cough reflex as means to clear the lungs.
The inhaled chemicals can also contribute to the breakdown of connective tissue fibers, especially elastin. The degeneration of the connective tissue can lead to emphysema, a form of chronic obstructive pulmonary disease (COPD).
Blood and Nerve Supply
The lungs have a dual blood supply. The pulmonary artery brings oxygen-poor blood from the right ventricle of the heart. This blood passes through the pulmonary capillaries, where some carbon dioxide will leave the blood and a large amount of oxygen will be acquired. The newly oxygenated blood enters the pulmonary veins and returns back to the left side of heart. The pulmonary circulation holds about 500 milliliters (ml) of blood, or about 10 percent of the body’s supply. About 75 ml of blood is in the pulmonary capillaries for gas exchange at any one time. The blood supply that nourishes the tissues of the lungs arrives through the bronchial artery, which branches off of the aorta and carries oxygen-rich blood to support the lung tissues. The bronchial supply anastomoses with the pulmonary vessels, and a mixture of blood leaves through the bronchial and pulmonary veins. Blood passes through the lungs at a rate equal to cardiac output, or about five liters per minute.
Blood pressure measured in the pulmonary circulation is less than it is in corresponding vessels of the systemic circulation. To some extent this decrease results from the decreased resistance found in this shorter pulmonary pathway. Under normal conditions, this filtrate is readily removed by the lymphatic vessels. Under conditions where left atrial pressure rises dramatically, such as in mitral valve stenosis or congestive heart failure, pulmonary capillary pressure will also rise, increasing the rate of capillary filtration. If the lymphatic system cannot keep up with the higher filtration rate, fluid will accumulate in the alveoli as pulmonary edema. This can interfere with the exchange of oxygen, leading to cyanosis, decreased activity tolerance, etc.
The pulmonary circulation responds to hypoxia differently than the systemic circulation. The systemic circulation dilates under hypoxic conditions causing an increased blood flow through the tissues. The arterioles of pulmonary circulation constrict selectively in cases of alveolar hypoxia. This constriction diverts blood to areas in the lungs that are better ventilated. This helps ensure adequate gas exchange.
Nerves from the pulmonary plexus enter the lungs at the hilus. These nerves contain a mixture of visceral sensory and autonomic nerve fibers that follow the bronchial tree and blood vessels. Parasympathetic nerve stimulation results in bronchoconstriction, constriction of the bronchioles, while sympathetic nerve stimulation results in bronchodilation, dilation of the bronchioles.
Pleura
Each lung is found in a pleural cavity bounded by the pleural membrane, a double sided membrane that contains a thin layer of pleural fluid. The visceral pleural is a mucus membrane that covers the lungs and folds over at the hilus. The folded membrane continues and becomes the parietal pleura, which lines the inner wall of the thoracic cavity. The space between the two membranes is called the pleural cavity or space. From 1 to 15 ml of pleural fluid is found on the facing surfaces of the pleural membranes. This fluid helps to lubricate the membrane surfaces so that the movement of the lungs during inhaling and exhaling does not cause frictional damage to the tissues. The fluid also lightly holds the two membranes together so that they move together as the chest wall expands and contracts.
Since pleural fluid is mainly a filtrate of plasma, the factors that affect the amount of pleural fluid production and removal are the same factors that govern interstitial fluid volumes in most regions of the body. The main factors include capillary hydrostatic pressure, capillary colloid osmotic pressure, the permeability (“leakiness”) of the capillaries, and the rate of fluid removal by the lymphatics. Under normal conditions, there is a slow but steady turnover of pleural fluid, but under certain conditions excess fluid can build up, and impede lung expansion. This condition is known as a pleural effusion and may be secondary to blockade of venous drainage by tumors, increased capillary permeability because of infections, etc.
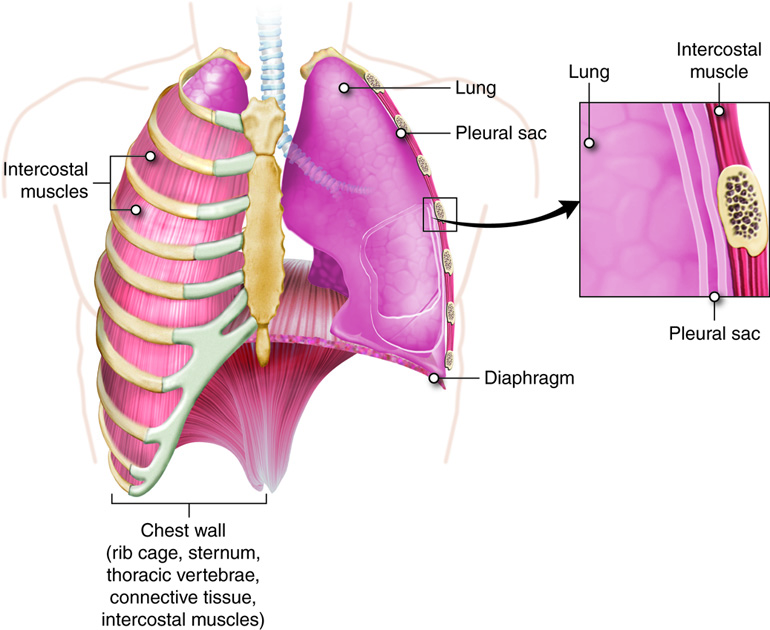
Respiratory Functions
The movement of gases from the atmosphere into the lungs and the cells of the body and back out again is called external respiration. External respiration includes ventilation, the bulk flow of air from outside the body into the repiratory system (inhalation) and the bulk flow of air from the respiratory system back to the outside (exhalation). Also included under the processes described as external respiration are the exchange of oxygen and carbon dioxide between the lungs and the blood, the transport of oxygen and carbon dioxide by the blood, and the exchange of gasses between the cells and the blood. The conduit for moving air into the lungs and then returning it to the external environment is referred to as the conducting zone (division). Gas exchange does not take place in the structures that make up the conducting zone. The conducting zone consists of a system of tubes that connect the nose and mouth to the alveoli. Air movement starts at the nose or mouth and continues through the pharynx, larynx, trachea, and bronchial tree, terminating at the structures of the respiratory zone (division) where gas exchange will occur.
The respiratory zone includes the very small respiratory bronchioles, the alveoli and the blood capillaries. It is critical that both the conducting and respiratory zones function adequately for the body to maintain homeostasis. Organization of conducting and respiratory zones will be the focus of this discussion.
The Conducting Zone
The conducting zone of the respiratory system brings gases into and out of the respiratory system. In addition, the organs and tissues along this path warm the incoming air to approximately body temperature, moisten the air to about 100 percent humidity, and begin filtering out any harmful microorganisms or particles that may be suspended in the inhaled air.
Temperatures too far below 37°C (98.6°F) can harm the delicate alveoli and inhibit enzyme function. Damaged aveoli, or improperly functioning enzymes could lead to decreased gas diffusion and subsequent homeostatic imbalances. For example, the inter-conversion of carbon dioxide and carbonic acid is catalyzed by the enzyme carbonic anhydrase. Similar to most other body enzymes, it loses efficiency at lower temperatures. If carbonic anhydrase is not functioning properly there could be an increase in carbonic acid levels. Since carbonic acid breaks down into bicarbonate ions and free hydrogen ions, there would be resulting decrease in pH leading to acidosis. The temperature in the respiratory zone is so important that a person who runs in cold weather is advised to put a scarf across their nose and mouth to help pre-warm the air. Otherwise, the tissues in the conducting zone may not be able to warm the air sufficiently to prevent damage to the system.
If the air in the respiratory zone is not near 100 percent humidity, the thin-walled alveoli can become dehydrated and may deteriorate. The alveoli are lined with a very thin layer of water and chemicals that prevent damage to the tissues and help to keep the alveoli open. If the air taken to the respiratory zone doesn’t have sufficient humidity (water vapor), the water layer lining the aveoli will evaporate resulting in damage to the structures and interference with respiration.
The hairs found at the entrance to the nose, along with the mucus and cilia found inside the nose help to trap and remove pathogens and particles taken in with air. Large particles can build up at the entrance of the nose where the hairs trap them. Coal miners, for example, will accumulate coal dust at the opening of their nostrils due to the action of these hairs. Smaller particles, such as some viruses and bacteria will be trapped in the mucus in the nose and the cilia on the epithelial cells will sweep them to the back of the pharynx. The combined action of the mucus and the cilia is referred to as the mucociliary escalator. Ultimately the mixture of mucus and particles will be swallowed and destroyed by the acid and digestive enzymes in the stomach. This process of trapping and removal of particles and microorganisms occurs throughout the conducting zone and through the first part of the respiratory zone.
The Respiratory Zone
A transition occurs in the composition of the epithelial lining at the end of the terminal bronchioles. The epithelial lining changes to simple cuboidal cells without any intermixed goblet cells. The walls of the tubes at this point become very thin and are called respiratory bronchioles. The loss of the goblet cells means that no mucus is secreted into the bronchioles at this point, but the cilia are still present to sweep away any mucus that should come down into them. Some portions of the respiratory bronchioles are not completely covered by cilia, and a number of phagocytic macrophages are found in this area to compensate for the loss of the cilia. Some gas exchange can occur through the walls of the respiratory bronchioles.
Respiratory bronchioles lead into the alveolar ducts, which still have smooth muscle and some elastic fibers in the walls. Alveolar sacs occur as clusters of aveoli (singular alveolus) sharing a common chamber along the alveolar ducts. It has been estimated that there are 300 million alveoli in the lungs. These alveoli provide a surface area of 27 square meters, or an area 25 feet by 30 feet, which is roughly the size of a three-car garage. Adjacent alveoli are interconnected through alveolar pores.
Pulmonary Ventilation
Pulmonary ventilation is the act of breathing and the first step in the respiratory process. Pulmonary ventilation brings in air with a new supply of oxygen and a very small amount of carbon dioxide from the atmosphere into the alveoli. This mixture then participates in external respiration, the exchange of oxygen and carbon dioxide between the alveoli and pulmonary capillary blood across the respiratory membrane. Internal respiration is the exchange of gasses between the tissues of the body and the blood, which provides oxygen for aerobic cellular respiration and removes carbon dioxide. Aerobic Cellular respiration refers to the intracellular use of oxygen and the generation of carbon dioxide waste through metabolic pathways.
Pressure
Breathing or ventilation, involves changes in pressure as a result of mechanical work.
The physical movement of air into the lungs is a result of the production of differences in total pressure between the interior (alveolar pressure) and exterior (atmospheric pressure) of the respiratory zone. Atmospheric pressure at sea level is typically 1 atmosphere or 760 mmHg, and this value will be used as the reference atmospheric pressure here. One atmosphere is the air pressure that would push a column of the mercury up in a thin tube a distance of 760 mm. Mercury is used because it is a liquid at room temperature, and its density changes very little over pressure and temperature ranges. In the process of ventilation, air moves down pressure gradients going from an area of higher pressure to an area of lower pressure. Assuming a person is at sea level, if his or her intrapulmonary pressure, the pressure in the alveoli, is less than 1 atm, air enters the lungs and fills the alveoli. If his or her intrapulmonary pressure is greater than 1 atm, then air moves out of the lungs into the environment.
There is another pressure often discussed in the mechanics of ventilation, intrapleural (sometimes just pleural) pressure. This is the pressure within the pleural sac (space). Intrapleural pressure is maintained slightly less than the pressure in the alveoli. This pressure difference aids in keeping the lungs slightly inflated at all times and ensures that they do not collapse when exhaling.
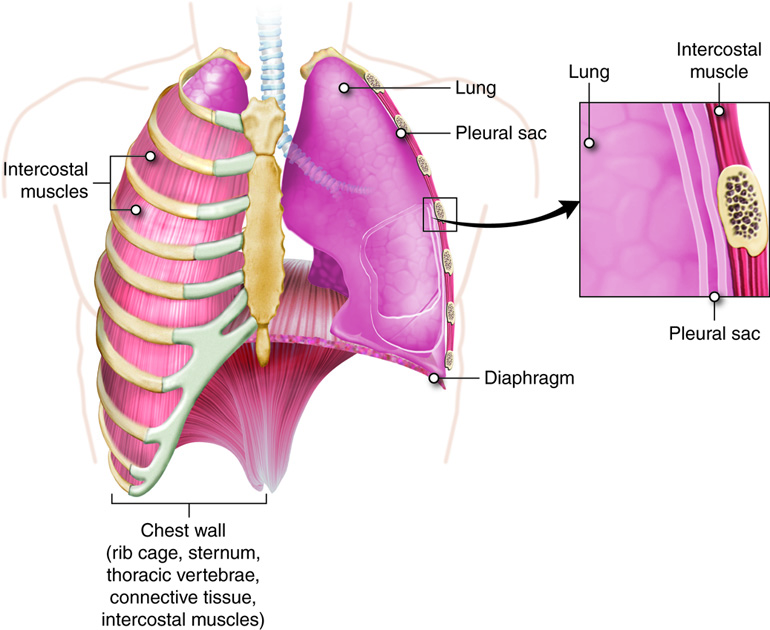
Inspiration and Expiration
Gases have a property of distributing to fill whatever size and shape container they occupy. If a closed container is made larger (an increase in volume), the total number of gas molecules will stay the same, but they will redistribute to fill the larger space. In doing so, they decrease their concentration (remember that concentration is a property of mass and volume). This increase in volume but not number of molecules of the gas leads to a corresponding decrease in the pressure exerted by that gas on the walls of the container. If the same closed container is made smaller, the concentration of gases increases( even though the actual number of gas molecules has not changed) and the pressure increases. This is an example of a physical law called Boyle’s Law.
The law is expressed mathematically as:
P1V1 = P2V2
where P = pressure, V = volume, 1 is the initial pressure and volume, and 2 is the resulting pressure and volume. Boyle’s Law states that, at a constant temperature, the pressure of a gas is inversely proportional with the volume of its container. Very simply, as volume goes up, pressure goes down, and as volume goes down, pressure goes up.
These properties become important for ventilation because the passage of air into and out of the lungs is controlled by the size of the lungs’ container, the thorax. The difference between the example above and the lungs is that the lungs are not a closed system (unless you have a closed glottis), with the opening to the lungs maintained through the conducting zone. But over very short periods of time Boyle’s law still applies. In this case, when we inhale, the movement of the diaphragm and the ribs increases the volume of the thorax. This immediately decreases the air pressure within the thorax, creating a pressure gradient between the atmosphere and the alveoli (the latter is called intrapulmonary pressure), and drawing air into the lungs. Air will enter until the atmospheric and intrapulmonary pressures are equal. However, the volume of the thorax remains larger than before the start of inhalation. To exhale, the thorax is allowed to decrease in volume (the ribs and diaphragm return to their original positions), increasing the intrapulmonary pressure and creating a gradient in the opposite direction resulting in the flow of air out to the atmosphere. Changing the size of the thoracic cavity would be impossible if the ribs were solidly attached to the sternum. If they were solidly attached, it would be like trying to move the sides of a birdcage. The costal cartilage allows the ribs to move and expand or contract the chest wall.
Inspiration
Inspiration is the act of inhaling. As stated above, inspiration occurs by increasing the volume of the thorax. This active process involves the use of chest and neck muscles. Resting inspiration is achieved mostly by movement of the diaphragm. The relaxed shape of the diaphragm resembles a shallow dome with the apex pointing toward the lungs, similar to the shape of an open umbrella. When the diaphragm contracts, it tends to flatten out, expanding the volume of the thorax in an inferior direction. Consequently, the intrapleural and intrapulmonary pressures decrease below atmospheric pressure resulting in air being pulled through the conducting zone and into the lungs. The external intercostal muscles work in conjunction with the diaphragm. The normal orientation of the ribs is around the side of the thorax and angled inferiorly to the sternum. When the external intercostal muscles contract, the ribs are pulled up, also expanding the thoracic cavity in a horizontal direction. In adults, ventilation occurs about 12 times a minute and moves roughly 500 ml of air during each breath.

A deep breath involves a greater shortening of the diaphragm and the external intercostal muscles plus additional contractions of other neck and chest muscles. The scalene muscles elevate the first two ribs. The sternocleidomastoid muscles elevate the sternum, and the pectoralis minor muscles help to elevate the third, fourth, and fifth ribs. Two physical conditions that interfere with inspiration are obesity and advanced pregnancy. In both cases, the abdominal organs are pushed against the diaphragm, restricting its downward movement and hindering the expansion of the thoracic cavity.
Expiration
Expiration is the act of exhaling. Resting expiration is a passive process. The muscles used during inspiration relax, and allow the chest wall and the diaphragm to move back to their original position, thus decreasing the volume of the thorax and forcing air from the lungs. The compression of the chest wall also aids in moving blood and lymph through the vessels that drain the lungs. Expiration becomes an active process when a more forceful exhale is required. The internal intercostal muscles pull the ribs down, helping to compress the chest. The external and internal oblique and transverse abdominal muscles press on the abdominal organs, which move them up against the diaphragm and force the diaphragm higher than it would normally go on relaxation, further decreasing the volume of the thoracic cavity.
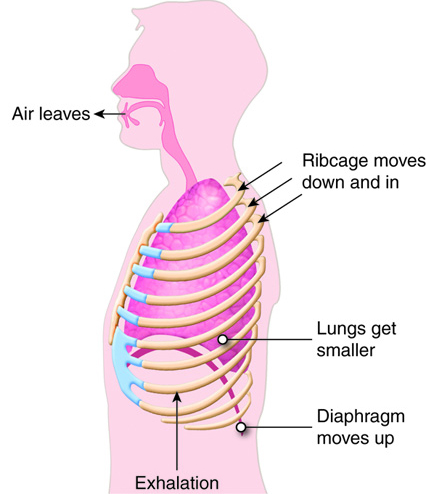
Airway Resistance, Alveolar Surface Tension, and Lung Compliance
One thing that works against the pressure gradient created by the expansion of the thorax is the resistance found within the conducting zone. Under normal conditions this resistance is quite low, and the airways have little trouble passing air between the atmosphere and the alveoli. The small amount of resistance found in the system stems mainly from the bronchioles, which are analogous to the arterioles of the vascular system. Both the bronchioles and the arterioles contribute a large portion of the resistance of their entire system, and both have smooth muscles in their walls that allow them to constrict or dilate. Under conditions of bronchiolar constriction (bronchoconstriction), resistance to airflow can increase dramatically. When this happens rapidly, it is classified as an acute asthma attack. The afflicted individual will need to generate much larger changes in intrapulmonary pressure to maintain a normal flow rate of air during breathing. Recall that if resistance increases an increased pressure gradient is necessary to maintain the same flow. To achieve this, the person uses the accessory muscles to breathe, and will appear to be “straining” as he or she does so. Treatment usually involves an inhaler containing a bronchodilator. Resistance will also be affected if the airways become narrowed by mucus (chronic asthma) or aspirated substances.
Along with the resistance of the airways, the compliance of the lung tissue also determines how much effort breathing requires. Compliance is a property that explains the relationship between a volume change and pressure change. Typically it takes a 2-3 cm of water change in pleural pressure to change volume in the lungs by 500 ml. This is a compliance of about 200 ml/cm H2O. The compliance of the lungs is dependent on the elasticity of the connective tissues of the lung as well as the alveolar surface tension. Remember that a thin liquid layer containing water as well as other molecules lines the interior lung surface. Because of its polar nature, water exhibits cohesion, such that it takes some effort to separate water molecules (you may have experienced the force of this cohesion if you ever belly flopped into the water). This surface tension is actually enough to collapse the alveoli each time a person exhales. In order to prevent this from happening, the type II alveolar cells make a combination of lipids and proteins that serves as a surfactant in the alveoli. A surfactant is a chemical that acts like a detergent breaking the surface tension of the water lining the alveoli. This action allows the alveoli to remain open when exhaling.
Compliance of the lung can be decreased either by fibrosis of the lung tissue creating a stiffening of the tissue (this can occur with conditions such as tuberculosis) or lack of surfactant (common in premature infants). Either way breathing becomes much more difficult, requiring more effort and sometimes mechanical ventilation.
Respiratory Volumes, Capacities, and Function Tests
Various lung volumes, capacities (capacities are combinations of lung volumes) and flow rates can be evaluated through a process called spirometry. The patient breathes in and out under controlled conditions, and the amount of air passing through the system, as well as the time it takes for air passage is measured. The values for these measurements depend on lung function and the size of the patient.
Respiratory Volumes | Description | Normal adult values |
---|---|---|
Tidal Volume (TV) | Volume of a resting breath | 500 mL/breath |
Inspiratory Reserve Volume (IRV) | Maximum volume that can be inhaled after a normal inhale | 1900 – 3300 mL |
Expiratory Reserve Volume (ERV) | Maximum volume that can be exhaled after a normal exhale | 700 – 1200 mL |
Residual Volume (RV) | Air left in the lungs after exhaling completely (i.e. after an ERV) | 1200 mL |
Dead Space | Air inhaled during breathing that stays in the conducting zone | 150 mL |
A normal, resting respiration is called the tidal volume (TV), and is typically about 500 ml per breath. Inspiratory reserve volume (IRV) is the amount of air that can be taken in “on top of” the tidal volume, with a deep breath. This ranges from 2,100 to 3,200 ml. The reverse of the IRV is the expiratory reserve volume (ERV), the amount of air that can be forcefully expelled after the tidal volume. The ERV range is from 1,000 to 1,200 ml. The conducting zone organs contain a volume of air that does not enter the respiratory zone (and therefore does not participate in gas exchange) called the dead space air. The amount of dead space air is typically 150 ml. The actual air will be moved during the next inspiration or expiration, but the volume of dead space air does not enter into the volumes involved in internal respiration.
The final normal respiratory volume is the residual volume (RV). This is the amount that is left in the lungs after the ERV is expelled. The RV is about 1,200 ml of air. The residual volume helps maintain open alveoli and is the air that the newly inhaled air is mixed with. No matter how hard a person exhales, there is always air left in the lungs. This remains true even after death. The only way to remove the residual air from the lungs is to replace it with something else.
Essentially this is what happens when a person drowns, water replaces residual air. If a pathologist needs to determine if a deceased person recovered from a body of water drowned or was dead before going into the water, he or she clamps off the trachea, removes the lungs, and places them in a bucket of water. If the person drowned, the lungs will sink because the residual air was replaced by the water. If the person was dead before going into the water, the lungs will float because the residual air is still there.
Several respiratory capacities can be calculated from the volumes listed above. The inspiratory capacity (IC) is the total amount of air that can be inhaled and is calculated by adding the tidal volume and inspiratory reserve volume. The functional residual capacity (FRC) is the amount of air remaining in the lungs at the end of a normal exhalation. This air continues to participate in gas exchange even after the person has exhaled. Functional residual capacity is calculated by adding together residual and expiratory reserve volumes. The vital capacity (VC) is the total exchangeable air in the system and is calculated by adding together tidal volume, inspiratory reserve volume, and expiratory reserve volume. Typically vital capacity is about 4,800ml of air. The total lung capacity (TLC) is the total of all lung volumes and is about 6,000 ml.
Respiratory Capacities | Description | Normal adult values |
---|---|---|
Inspiratory Capacity (IC) | TV + IRV | 2400 – 3800 mL |
Functional Residual Capacity (FRC) | RV + ERV | 1800 – 2200 mL |
Vital Capacity (VC) | TV + IRV + ERV | 3000 – 4600 mL |
Total Lung Capacity (TLC) | TV + IRV + ERV + RV | 4200 – 6000 mL |
Besides volumes and capacities, flow rates are often measured to assess a person’s lung function. Flow rates are important because although changes in airway resistance will not usually change volumes, they will affect the rate of air movement through the system. To assess flow rates, the individual takes as deep a breath as possible (gets to VC) and then exhales maximally and as quickly as possible. The time it takes to bring the lung volume down to RV provides information about airway resistance. Flow rates are used to assess asthma and related conditions.
Lung volumes and flow rates can be used to differentiate between obstructive and restrictive pulmonary diseases. Obstructive pulmonary disorders are those that increase the resistance to airflow, thus increasing the time it takes to move air in and out of the lungs. Examples of obstructive disorders include bronchitis and asthma. Restrictive pulmonary disorders are those that affect the compliance of the lung or affect the ability of the chest wall to expand and relax normally. Such disorders lead to lower lung volumes than would be expected based upon a person’s size. Examples of restrictive pulmonary disorders include those that lead to structural or functional changes in the lung tissue (tuberculosis, fibrosis) or those that impede normal muscular function (such as muscular dystrophy) or function of motor nerves (such as multiple sclerosis and ALS).
Respiratory Structures and Functions Summary
Structure determines function. To understand how the respiratory system performs its function it is essential to examine the anatomical structures of the system. Previously we examined the microscopic components of the respiratory system. Now we will places those components into the gross anatomy of the upper and lower respiratory tracts. After examining the structure we discussed how these structures perform the functions of the respiratory system and how the efficiency of these functions is measured.
- Entrance to the upper respiratory tract is through the nose or the mouth.
- The nasal cavity contains structures such as hair, sebaceous glands and mucus that aid in preventing microorganisms from entering.
- The nasal cavity also contains olfactory epithelium for the sense of smell.
- The conchae of the nose contain mucus glands and blood vessels to aid in humidifying and warming the air.
- The paranasal sinuses are hollow areas that make the skull lighter and also moisten and warm incoming air.
- Next the air passes into the pharynx which is divided into three areas and connects to the larynx.
- The nasopharynx is the superior portion of the pharynx and only acts as a passageway for air.
- The oropharynx extends from the soft palate to the epiglottis, and is covered in stratified squamous epithelia to protect it from the friction of food as it is swallowed.
- The laryngopharynx is the third and shortest portion of the pharynx. It extends from the epiglottis and ends above the esophagus. It is lined with stratified squamous epithelia to protect it from the friction of food as it is swallowed. Both the oropharynx and the laryngopharynx are passageways used to transport both air and food.
- The tonsils are patches of lymphatic tissue that help protect the body against pathogens by filtering them from the air.
- Pharyngeal tonsils (adenoids) are located in the nasopharynx
- Tubal tonsils protect the auditory tubes and middle ear.
- Palatine tonsils are located in the oropharynx
- Lingual tonsils are located under the tongue.
- The path of air then continues from the pharynx to the larynx which extends from hyoid bone to the trachea. At the juncture of the pharynx and the larynx air and food are directed into the larynx (and then trachea) and esophagus respectively.
- The glottis or opening to the larynx and thus the passageway to the trachea is blocked by the epiglottis when swallowing.
- The vocal cords are located in the larynx and used for speech
- The larynx also consists of nine cartilage structures that we use as anatomical landmarks. They function to maintain an open airway. The cartilages include the thyroid cartilage sometimes known as the Adam’s apple, the inferior thyroid cartilage (punctured when a tracheostomy is performed). The paired arytenoid, cuneiform, and corniculate cartilages.
- Although the lower respiratory tract begins at the larynx, most of the tract consists of the trachea, the bronchi and bronchioles, the alveoli and the lungs.
- The trachea, known as the windpipe, extends from the larynx to the fifth thoracic vertebrae. It is composed of C-shaped cartilage that maintain an open airway. The trachea consists of three layers of tissue the mucosa, submucosa and adventitia.
- The bronchial tree is next and is a series of passageways that grow smaller and smaller as they extend into the lungs. The cartilage plates are not present in the bronchioles. Plates of cartilage help maintain the opening of the passageways. As the size of the passageway decreases so does the number of plates. The respiratory bronchioles are the smallest and end in the alveolar sacs where gas exchange will occur.
- The lungs contain elastic connective tissue that allow them to expand and recoil during ventilation.
- Anatomical reference points of the lungs include 1) the hilus, the entry point for blood vessels, the primary bronchi and nerves 2) the apex of the lung, the most superior portion of the lung, and 3)the base of the lungs, located above the diaphragm.
- There is a right and a left lung. The right lung consists of three lobes while the left lung only has two lobes because of the location of the heart.
- Each lobe is divided into many lobules each of which contains a terminal bronchiole and its respiratory bronchioles and associated alveoli.
- The lungs have a dual blood supply. The pulmonary circuit brings deoxygenated blood to the lungs to release carbon dioxide and pick up oxygen to return to the heart. The systemic circuit brings oxygenated blood to feed the tissue of the lungs.
- The lungs are surrounded by the visceral pleural which is a mucus membrane and then the parietal pleural that attaches to the walls of the thoracic cavity.
- The space between the membranes is the pleural space and contains pleural fluid that can be examined for signs of infection.
- Pleural effusion can be caused by different conditions so the fluid is checked to see if it is exudate with increased proteins or transudate with normal protein levels.
- The primary function of the respiratory system is the movement of gases from the atmosphere into the lungs and to provide oxygen to the body while ridding the body of carbon dioxide.
- External respiration includes ventilation and the movement of gases between the lungs and the blood and between the blood and the systemic tissues.
- The passageway for air moving into and out of the body can be divided functionally into two divisions: the conducting zone and the respiratory zone which allows for air to diffuse from the lungs into the blood.
- The conducting zone brings air into and out of the lungs. As the air passes through the conducting zone it is warmed to body temperature, humidified and microorganisms are filtered out.
- The temperature and humidity must be correct so the delicate respiratory zone is not damaged.
- The respiratory zone consists of the respiratory bronchioles and the alveolar sacs and is the location of gas exchange between the lungs and the blood.
- Pulmonary ventilation is the act of moving air into and out of the lungs and is the first step of external respiration.
- Pressure plays a huge role in moving air into and out of the lungs. Air follows pressure gradients moving from an area of high pressure to an area of low pressure.
- Air will move into the lungs if the alveolar pressure is less than the atmospheric pressure and air will move out of the lungs if the alveolar pressure is higher than the atmospheric pressure.
- Intrapleural pressure is the pressure within the pleural space and it will help keep the lungs slightly inflated to prevent collapse during exhalation.
- Pulmonary ventilation includes both inspiration and expiration. To understand how air moves into and out of the lungs, it is important to understand the physical laws that govern molecular movement.
- Boyle’s law states that initial pressure times initial volume is equal to the resulting pressure times the resulting volume.
- This means as volume goes up pressure goes down and as volume goes down pressure goes up.
- During inhalation the diaphragm and the external intercostal muscles of the ribs contract and move to increase the volume of the thorax resulting in a decrease the intrapulmonary pressure and causing air to move in.
- During exhalation the diaphragm and the external intercostal muscles of the ribs relax and decrease the volume of the thorax resulting in an increase in the intrapulmonary pressure and causing air to move out.
- During forced inspiration contraction of additional muscles of the neck will allow more air to enter.
- During forced expiration muscles of the abdomen help force the diaphragm up further decreasing the volume of the thoracic cavity and increasing the volume of air exhaled.
- Additional factors that affect the pressure gradient include airway resistance, alveolar surface tension and lung compliance.
- Smooth muscle of the bronchial tubes can constrict or dilate to alter the airway resistance.
- Conditions such as asthma can cause an increase in resistance that will result in a person needing to use accessory muscles to maintain adequate air flow.
- Compliance will also affect the amount of effort required for breathing. Compliance depends on the elasticity of the connective tissue in the lungs as well as the alveolar surface tension.
- Compliance can be decreased by fibrosis of the lung tissue or by the lack of surfactant.
- To evaluate the efficiency of a person’s inhalations and exhalations the process of spirometry is used.
- A person breathes in and out under controlled conditions and the amount of air and the flow rate can be measured.
- The respiratory volumes include: Tidal Volume, Inspiratory Reserve Volume, Expiratory Reserve Volume, Residual Volume, and Dead Space.
- The Respiratory Capacities are two or more respiratory volumes added together. They include: Inspiratory Capacity, Functional Residual Capacity, Vital Capacity, Total Lung Capacity
- Flow rates can also be used to assess a person’s lung function because there can be changes in the air flow that do not affect the volumes.
The lungs function to provide the entire body with the oxygen required to convert nutrients to energy. The lungs also need to be able to remove excess carbon dioxide from the body. To assess a person’s lung function, a person can breathe into a spirometer. The patient’s Respiratory Volumes and Capacities and flow rate can be measured. These measurements will vary based on a person’s size, age and gender. Restrictive lung disorders such as tuberculosis and obstructive disorders such as bronchitis can impede normal airflow and can be differentiated using spirometry.