56 Digestive Levels of Organization
In order to get the various materials needed for energy production and anabolic metabolism in all body cells, our body has to exchange nutrients, water and electrolytes from the environment. However, the substance taken into the space of the GI tract is not always in the form that can be absorbed, so it must first be broken down. Various secreted chemicals are critical to accomplishing this digestion. These ingested macromolecules are digested in different regions of the GI tract, requiring that the mixture of material be moved through the digestive system in a controlled way. Once it has been digested to a basic chemical structure, the material can be absorbed into the blood or lymph. At that point, the other body systems integrate to distribute these absorbed nutrients, fluids and electrolytes through the body as needed. Any material remaining in the GI tract as waste will then be removed from the body by defecation.
To understand these system-level functions, you will be further exploring structures and processes occurring at all levels of organization. Some examples of major digestive structures assigned to their structural level of organization of the digestive system include:
- Chemical level– sodium, potassium, chloride, bicarbonate, and phosphate ions and hydrochloric acid
- Macromolecular level– enzymes of digestion, bile, and the nutritive molecules such as carbohydrates, protein, lipids and nucleic acids
- Cellular level– mucosa cells, secretory cells, and immune cells
- Tissue level– epithelial, connective, and smooth muscle tissues
- Organ level– gastrointestinal tract and accessory organs
- Organ System level– integration of organs for nutrient and waste movement, digestion and absorption
Chemicals and Macromolecules
Chemical Digestion
The digestive system breaks down ingested material into absorbable components (nutrients) that the rest of the body can use to maintain adequate function. We require a diverse set of nutrients to support energy production, carry out metabolism and maintain structure.
Chemical digestion generally, breaks down large food molecules into their respective chemical building blocks, called monomers, that can be absorbed. The enzymes responsible for chemical digestion are released by both intrinsic glands found in the gastrointestinal tract and the accessory glands which secrete molecules into the gastrointestinal tract.
Hydrolysis refers to the breakdown of a chemical where water breaks a covalent bond. Hydrolysis can happen spontaneously, but the breakdown of food into macromolecules and monomers occurs by enzymatic hydrolysis – where the hydrolysis reaction catalyzed by an enzyme. Hydro- is part of the name because a water molecule is added to each molecular bond that is broken, or -lysed. More specifically, hydrolysis is a method of degradation which splits a molecule of water to help break chemical bonds in a larger molecule. Usually an H+ is attached to one of the components and an OH- group to the other. Hydrolysis reactions are important for many other physiologic processes in the body in addition to the breakdown of food molecules.
Nutritive Molecules
The nutritive organic compounds in our food includes carbohydrates, proteins and lipids. These molecules are digested, then absorbed, and reassembled into macromolecules or used as fuel for metabolism in the body. The process of breaking down these macromolecules involves splitting into smaller molecules using water molecule, thus the name hydrolysis (“hydro” = water; “lysis” = splitting). The reverse reaction, called dehydration synthesis, can build macromolecules from the absorbed building blocks. Enzymes can speed up the rate of these reactions.
Carbohydrates
Our daily food intake usually includes from 200 to 600 grams of carbohydrates. Carbohydrates are primarily used for quick energy, but some are also used to create important signaling and structural molecules. The monomers of carbohydrates, monosaccharides (simple sugars), are absorbed easily and can therefore be a source of quick energy for the body when we consume them in this form. Glucose, galactose, and fructose are the three common monosaccharides in our diet.
Disaccharides are two monosaccharides bound together and include sucrose (table sugar), lactose, and maltose. Polysaccharides are long chains of monomers and include polymerized glucose in different forms including glycogen, which is the stored form of glucose in our bodies, and starch, a polysaccharide of glucose molecules that comes from plant sources.
In many places around the world, starch accounts for the largest portion of digestible carbohydrates in the diet, with the addition of some glycogen, disaccharides and monosaccharides. There are other polysaccharides in our diet, like cellulose, but our bodies do not produce enzymes that can break them down, so they are indigestible. While indigestible polysaccharides do not give us any nutrients, they do provide bulk (fiber) that helps propel food through the digestive system.
Lactose Intolerance
Individuals with lactose intolerance are unable to metabolize lactose, a sugar found in milk. The problem is usually caused by a lack of the enzyme lactase, which is required to break down lactose in the lining of the small intestine. Without breakdown in the small intestine, lactose flows into the large intestine where bacteria metabolize lactose in a process called fermentation. In fermentation, gasses are produced that cause abdominal bloating. Sugars and fermentation products also cause large amounts of water to enter the large intestine, leading to loose stool. Most infants are born the enzyme lactase, and as adults lose the ability to produce this enzyme.
Proteins
We get protein when we eat meat, seafood, eggs, beans, nuts and soy products. USDA recommends 5 to 6 ounces of protein in diet per day, although children need less. This dietary protein is usually in the form of polypeptides and must be digested into its amino acid building blocks for absorption. There are numerous enzymes that break large proteins into smaller peptides and then into amino acids. Amino acids are then absorbed from the digestive system into the circulatory system where they are delivered throughout the body. Once amino acids have entered cells throughout the body, they are bonded together to make proteins needed for cell function. As a last resort, proteins and amino acids can also be used as energy; they are metabolically converted to glucose before they are used as energy sources.
Lipids and Fats
Dietary lipids include fats and oils. While not considered a USDA food group, oils contain some essential nutrients and are recommended as part of a healthy diet, although only in small amounts. Solid fats have more saturated and trans-fatty acids and are considered empty calories when included in a diet because they add calories but not needed nutrients. Most dietary lipids are in the form of triglycerides, with one glycerol molecule and three fatty acids bound together. Lipids are processed by enzymes secreted from the pancreas (with some enzymes from the stomach and saliva) and are then solubilized for absorption by salts secreted in bile (from the liver). These steps prepare them for absorption in the small intestine. Like proteins, ingested lipids are broken down into smaller parts for absorption and then are either metabolized to make energy or are use to make cellular structures including cell membranes. Lipid absorption is also required for absorption of some fat-soluble vitamins.
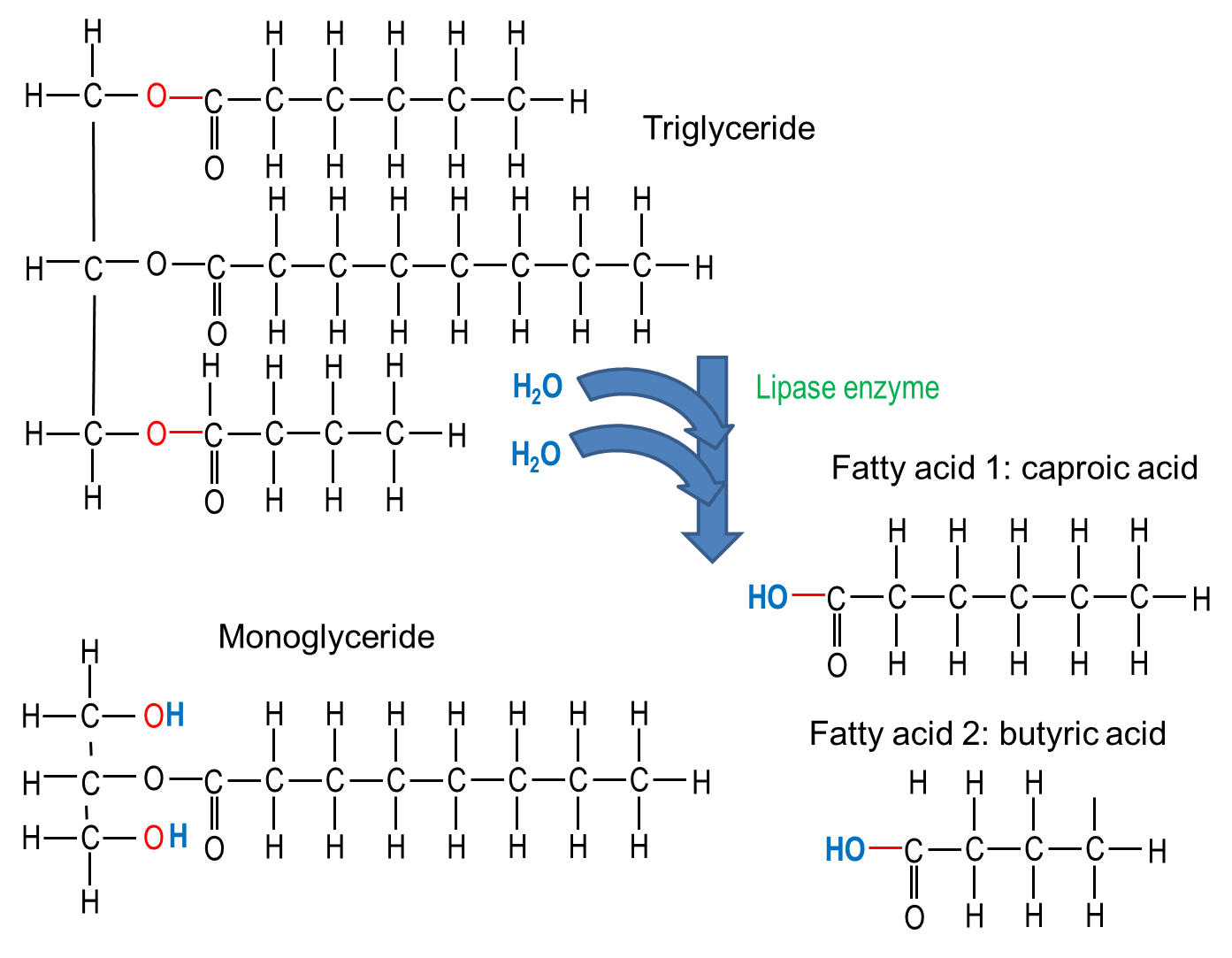
Nucleic Acids
When we eat any plant or animal foods, we are able to digest the DNA or RNA nucleic acid that was in their cells. The fundamental unit of nucleic acids is the nucleotide, with a phosphate group, a pentose sugar, and one of 5 nucleic bases. The pancreas produces and releases into the small intestine two enzymes to break down either DNA (deoxyribonuclease) or RNA (ribonuclease) into individual nucleotides. Brush border enzymes of the cells lining the small intestine further digest the nucleotide into its molecular components for absorption.
Digestive Molecules
Saliva
Some salivary glands are always secreting saliva. Intrinsic salivary glands of the mouth secrete small amounts of saliva, usually just enough to moisten the mucous membranes and clean the mouth and teeth. The accessory salivary glands are exocrine organs under autonomic nervous system control with ducts leading into the mouth. Secretion increases when there is food in the mouth, as well as through a reflex when food is smelled, seen or thought about (you have probably heard about Pavlov’s experiment with the salivating dog and bell). Saliva is needed to moisten and lubricate the food and begin its chemical breakdown.
Saliva is mainly water, which dissolves chemicals in the food. Only dissolved chemicals can activate the different kinds of taste receptor cells on the tongue, palate and other parts of the mouth and pharynx. Mixed in with the water, saliva also contains mucus, various electrolytes typically found in blood plasma, as well as some digestive molecules, metabolic waste products and immune molecules. Each of these contributes to the various functions of saliva.
Bicarbonate and phosphate ions help maintain the pH of saliva as neutral or slightly basic (average 7.4 pH). This not only helps protect the teeth from acidic substances eaten and plaque bacteria that grow best in an acidic environment, but also maintains the optimal pH for the enzyme amylase. Salivary amylase starts breaking complex carbohydrates eaten, like starch, into sugars. This enzymatic activity will stop once the mixture of food and saliva, called a bolus, is swallowed and reaches the acidic environment of the stomach. Salivary lipase is another enzyme in saliva that breaks down dietary lipids, but it is activated when it reaches the acidic gastric juice with its optimal pH being much more acidic than the salivary pH.
In addition to the water of saliva, mucus in the saliva is composed of glycoproteins and helps moisten the food, so it can be manipulated into a mass for swallowing. It also helps lubricate the movement of food through the pharynx and esophagus by peristalsis. Lysozyme antimicrobial enzymes and antibodies (Immunoglobulin A) in the saliva help combat pathogens that might enter the body with food or drink. Like the sweat glands, salivary glands play a role in some metabolic waste excretion, so urea and ammonia are also found in saliva.
Enzymes and Acids of the Stomach
Gastric glands produce hydrochloric acid (HCl), enzymes, and intrinsic factor. HCl is responsible for the high acidity (pH 1.5 to 3.5) of the stomach contents. The acidity directly kills a lot of the bacteria we ingest with food and helps denature proteins and substances found in plants. In the presence of gastric juice’s low pH, the inactive enzyme, pepsinogen, is modified to become the active protein enzyme, pepsin. Protein enzymes of the digestive system are produced and secreted in an inactive form, to be activated only in the lumen, thus preventing digestion of the cells themselves. The glycoprotein intrinsic factor is necessary for the absorption of vitamin B12 later in the small intestine.
It is amazing that cells of the body can produce acids that normally would destroy the cell itself. Strong acids are also produced in cells in the immune system (to break down foreign organisms) and the skeletal system (to break down mineralized bone). High levels of acidity in the wrong places can be very destructive to living cells. Our body has several mechanisms to control pH.
In the stomach cell, the enzyme carbonic anhydrase converts one molecule of carbon dioxide and one molecule of water indirectly into a bicarbonate ion (HCO3–) and a hydrogen ion (H+). In the stomach ion exchange is used to move H+ ions out the cells and into the lumen of the stomach.
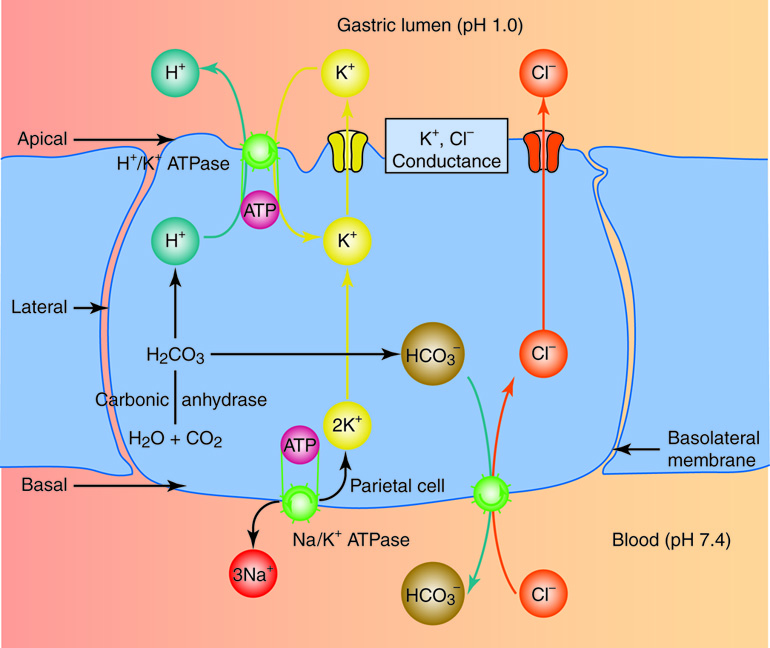
The bicarbonate ion (HCO3–) is then exchanged for a chloride ion (Cl–) on the basal side (away from the lumen) of the cell. Potassium (K+) and chloride (Cl–) ions diffuse into the secretory region of the cell called the canaliculi. Then the potassium is exchanged in this region for hydrogen ions via a H+/K+ ATPase. The hydrogen ions, which are millions of times more concentrated in this region of the cell than any other, are then pumped from the canaliculi into the lumen of the stomach. The following video illustrates this process.
Production of Hydrochloric Acid in the Stomach
Explanation of how the stomach produces hydrochloric acid without the lumen’s cells being harmed in the process.
Mucosal Barrier
Once the hydrochloric acid is produced in the stomach lumen, this creates a low (acidic) pH that could potentially destroy the cells in the mucosal layer of the stomach. However, there are several structural and functional components that are part of an effective mucosal barrier that protects the stomach cells and prevents the stomach from digesting itself. The thick mucus layer produced by gastric mucus cells has lots of bicarbonate ions that will buffer the effect of the acid in the lumen. This stable, pH neutral mucus layer also provides a barrier to the now activated pepsin enzymes in the lumen as well. The surface gastric epithelial cells under the mucus layer have tight cell-to-cell junctions that would prevent transcellular reabsorption of hydrochloric acid from the lumen. Like epithelial tissue elsewhere in the body, these gastric surface cells are replaced continually by gastric stem cells that are stimulated to divide more rapidly if there is tissue damage from the gastric juice. Prostaglandins are chemical messages involved in cell inflammation response to injury. Prostaglandins produced in the stomach stimulate production of gastric mucus and bicarbonate and promote tissue healing. When the mucosal barrier breaks down and tissue destruction reaches into the deeper connective and muscular layers of the stomach, this is called a peptic or gastric ulcer.
Ulcers: When the Mucosal Barrier Breaks Down
Aspirin and other non-steroid anti-inflammatory drugs (NSAIDs) are known to increase the risk of gastric ulcers. NSAIDs break down the mucosal layer and reduce secretion of bicarbonate ion, but even when they are enteric coated to protect the cells from contact, they increase the risk of gastric ulcers.
The acidity of gastric juice destroys many, but not all bacteria. There is one bacterium, Helicobacter pylori, adapted to live in the stomach mucus layer. Most people have this bacterium in their stomach with no symptoms, but in some people the bacterium is linked to increased incidence of gastric ulcers. There is still uncertainty about why only some people are affected this way. The bacterium uses the enzyme urease to convert urea to ammonia that helps neutralize acid in the mucus layer locally. If the mucus becomes too acidic for the bacterium, it can also induce an inflammatory response that lowers the gastric juice acidity. This immune response and associate release of free radicals may stimulate the formation of the ulcers in affected individuals. Once the causal link between ulcers and H. pylori was discovered, a new treatment for ulcers was implemented and the number of gastric ulcers in developed countries fell sharply.
There is evidence to suggest that the H. pylori should be considered to be colonizing the stomach, like other GI tract microbiota, rather than infecting the stomach because it does have some protective effects. As the incidence of gastric ulcers fell in developed countries due to the use of antibiotics, the incidence of esophageal reflux and cancer increased. Research has shown that the presence of H. pylori is inversely related to the incidence of not only GERD and esophageal cancer, but also childhood diarrhea, IBS, asthma and even tuberculosis. So there are interesting interactions between this bacterium and its human host that need further study.
Control of Gastric Secretions
The secretion of gastric juice is controlled by nerves, hormones, and the presence of products of digestion. Stimuli from smell, sight and taste as well as food in the oral cavity, stomach, and small intestine activate or inhibit gastric juice production. This is why the three phases of gastric secretion are called the cephalic, gastric, and intestinal phases. However, once gastric secretion begins, all three phases can occur simultaneously.
The cephalic (reflex) phase of gastric secretion, which lasts just a few minutes, takes place before food enters the stomach. The smell, taste, sight, or thought of food triggers this phase. For example, impulses from receptors in taste buds or the nose are relayed to the brain, which returns signals that increase gastric secretion to prepare the stomach for digestion. This enhanced secretion is a conditioned reflex, meaning it occurs only if we like or want a particular food. Depression and loss of appetite can suppress the cephalic reflex.
The gastric phase of secretion lasts three to four hours and is set in motion by local neural and hormonal mechanisms triggered by the entry of food into the stomach. For example, stomach distension activates stretch receptors that stimulate parasympathetic neurons to release acetylcholine, which then provokes increased secretion of gastric juice. Partially digested proteins, caffeine, and rising pH stimulate the release of gastrin from enteroendocrine G cells, which in turn provokes parietal cells to increase their production of HCl and increase motility through the stomach by altering sphincter function. The presence of proteins in the stomach raises pH, which stimulates the increased gastrin and HCl release needed to create an acidic environment for protein digestion.
GERD
If this control of gastric juice secretion is not working properly and too much hydrochloric acid is produced, conditions such as Gastroesophageal Reflux Disease (GERD) can occur. The acidic pH irritates the lining of the esophagus near the cardiac or lower esophageal sphincter and can lead to esophageal cancer. Antacids provide only temporary relief. Some drugs known as histamine-2 antagonists (H2-blockers) or proton-pump inhibitors (PPIs) may be needed to control the amount of stomach acid produced. However, the two drugs act on different parts of the parietal cell metabolism.
Intestinal Juice and Accessory Secretions
Pancreatic Juice in Digestion
The pancreas produces about 1.2 to 1.5 quarts (1.1 – 1.4 liters) of pancreatic juice each day. This clear, colorless liquid is mostly water, along with some salts, sodium bicarbonate, and several digestive enzymes. Sodium bicarbonate is responsible for the slight alkalinity of pancreatic juice (pH 7.1 to 8.2). The sodium bicarbonate buffers the acidic gastric juice which has arrived in the small intestine from the stomach, inactivates pepsin from the stomach, and creates an optimal pH for the activity of the digestive enzymes in the small intestine.
Just as the stomach produces pepsin in its inactive form (as pepsinogen), the pancreas creates and produces its protein-digesting enzymes as zymogens. These zymogens (trypsinogen, procarboxypeptidase, and chymotrypsinogen) are activated in the duodenum. If produced in their active forms, they would digest the pancreas itself. The intestinal enzyme enteropeptidase converts trypsinogen to the active trypsin. Trypsin also converts pancreatic enzymes procarboxypeptidase and chymotrypsinogen to their active forms, carboxypeptidase and chymotrypsin. Some pancreatic enzymes, including amylase, lipase, and nuclease, are secreted in their active form, but their optimal activity requires interactions with ions or bile in the intestinal lumen.
Bile
Bile is another accessory organ contribution to the GI tract lumen. Bile is made by the hepatocytes of the liver, but stored in, concentrated, and released from the gall bladder. Unlike pancreatic juice that is produced and released as needed, bile is produced in small quantities by the liver continuously. The gall bladder stores it for release into the small intestine as needed.
Like saliva, bile is a mixture of mainly water with many substances, including electrolytes, bile pigments, bile salts and lipids (including the phospholipid lecithin and cholesterol). The bile pigments include bilirubin and biliverden, which are waste by-products from the destruction of the hemoglobin in aged or damaged red blood cells destroyed in the liver. These wastes are then eliminated from the body with the feces and contribute to its color. In addition, cells lining the bile duct secrete bicarbonate ions into the bile. However, it is the lecithin and bile salts produced from cholesterol that have a digestive function related to dietary lipids.
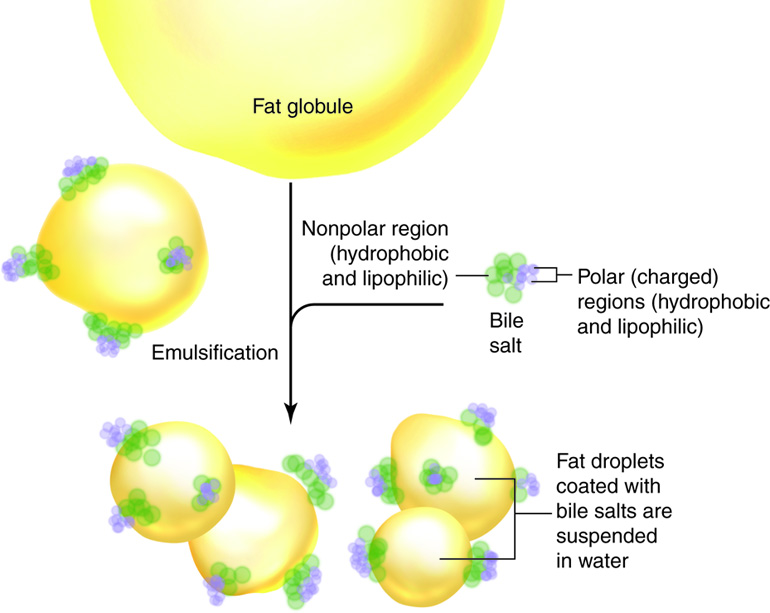
Recall that phospholipids in the cell membrane have a hydrophilic and hydrophobic portion. Bile salts are also amphiphilic. When they are released into the small intestine as part of the bile, the hydrophobic/lipophilic portion of the molecule is attracted to fat globules in the lumen, while the hydrophilic/lipophobic portion is attracted to the water in the digestive juice mixture. This chemical attraction and mechanical mixing breaks large fat globules into smaller droplets suspended in the fluid. The bile salts prevent the droplets from rejoining and thus increase the surface area of the lipid exposed to lipase enzymes; but the bile salts don’t actually digest the lipid into fatty acids and monoglycerides. This process is called emulsification.
Emulsification
Think of what happens when you mix oil and vinegar for a salad dressing. You can mechanically shake it up to produce smaller fat droplets suspended in the vinegar, but if left to sit, the two layers separate again. Lecithin from egg yolks is the emulsifier added to mayonnaise, which is a mixture of oil in vinegar or lemon juice that has been emulsified.
Once the lipids have been digested, the bile salts are still attracted to the lipid and form small micelles. These micells come in contact with the small intestine cell membranes, releasing and facilitating absorption of its fatty acids, monoglycerides and lipid-soluble vitamins. The bile salts are later reabsorbed themselves in the large intestine and recycled through the hepatic portal vein back to the hepatocytes, in what is called the enterohepatic circulation. Soluble fiber in the diet, such as psyllium, interferes with the reabsorption of these bile salts. As a result, the hepatocytes metabolize new bile salts using cholesterol from the blood, helping to lower LDL cholesterol.
There are also other mechanisms by which both soluble and insoluble fiber affect the body’s cholesterol metabolism and balance. The liver also excretes excess cholesterol in the bile. When the bile becomes supersaturated with such excess cholesterol, one of the three kinds of bile stones may form and obstruct the bile ducts. Not surprisingly, this type of gallstone is often associated with obesity. The absence of bile salts in bile results in a condition called steattorhea, where fats remain mainly undigested in the feces and can lead to diarrhea and fat malabsorption. This condition can result from liver damage or be a side-effect after the gallbladder is removed (cholecystectomy).
Intestinal Juice
The intestinal glands at the base of villi produce intestinal juice, a mixture of water and mucus. Each day, about one to two quarts (1-2 liters) are secreted. The mucus in intestinal juice is produced by both the duodenal glands and the goblet cells of the mucosa. The difference is that the mucus produced by the duodenal glands is alkaline, while goblet cell mucus is typically neutral. Progressing along the jejunum and into the ileum, goblet cells become more abundant. Secretion of intestinal juice is primarily stimulated by distension of the small intestine or the irritating effects of hypertonic or acidic chyme on the intestinal mucosa. The mucus also protects the surface cells from abrasion as the food moves through the lumen. Although the intestinal juice mucus secreted doesn’t have a direct digestive function, it does play an important role as a substrate for digestion. Extending into the mucus, but embedded in the cell membrane of intestinal villi cells, are various enzymes. These cell membrane surface enzymes are very important for the final digestion of organic substances before absorption.
Overview of Chemical Digestion
Carbohydrate Digestion
The mouth is where the chemical digestion of starch and possibly glycogen begins. Salivary amylase acts to break down the polysaccharide starch into the disaccharide maltose, the trisaccharide maltotriose, and short chains of glucose called α-dextrins.
Pancreatic amylase in the small intestine continues the chemical digestion of starch and other digestible carbohydrates that have not been broken down into maltose, maltotriose, and α-dextrins by salivary amylase. After starch has been broken down into smaller fragments by salivary or pancreatic amylase, the brush-border enzyme α-dextrinase starts working on the resulting α-dextrins, breaking off one glucose unit at a time. The disaccharides sucrose, lactose, and maltose are not digested until they enter the small intestine. Here, three brush-border enzymes hydrolyze them into monosaccharides. Sucrase splits sucrose into one molecule of fructose and one molecule of glucose; lactase breaks down lactose into one molecule of glucose and one molecule of galactose; and maltase breaks down maltose and maltotriose into two and three glucose molecules, respectively. The digestive system can then absorb monosaccharides.
Protein Digestion
The digestion of protein starts in the stomach, where pepsin breaks down proteins into peptides. In the small intestine, trypsin and chymotrypsin hydrolyze polypeptides into smaller peptides, and elastase fragments whole proteins into peptides. The small peptides are then broken down into monomers. Carboxypeptidase cleaves a single amino acid from the carboxy terminus of a small peptide, whereas aminopeptidase cleave an amino acid from the amino terminus. Dipeptidase splits dipeptides in the middle, liberating two amino acids.
Lipid Digestion
Triglycerides and their breakdown products do not dissolve in water. Before they can be digested in the watery environment of the small intestine, large lipid globules must be separated into smaller lipid globules, a process called emulsification, which is aided by the presence of bile salts. Recall that bile salts facilitate emulsification of large lipid globules into small lipid globules of about 1 µm in diameter.[link to bile salts] This emulsification greatly increases the surface area to volume ratio of fat globules, which allows pancreatic lipase to access more lipid molecules.
The three lipases involved in the digestion of triglycerides and phospholipidsare lingual lipase (in the saliva), gastric lipase, and pancreatic lipase. Pancreatic lipase in the small intestine does most of the lipid digestion. Pancreatic lipase breaks down triglycerides into fatty acids and monoglycerides. The fatty acids include both short-chain (less than 10 to 12 carbons) and long-chain fatty acids.
Nucleic Acid Digestion
Nucleic acids, including DNA and RNA, are in the cells of all once-living things which we may ingest. There are two nucleases in pancreatic juice – deoxyribonuclease, which digests DNA, and ribonuclease, which digests RNA. The nucleotides that are the products of this digestion are further broken down by intestinal brush-border enzymes (nucleosidases and phosphatases) into pentose sugars, phosphates, and nitrogenous bases, which can be absorbed through the GI tract wall.
Enzyme | Source | Food | Product |
---|---|---|---|
Saliva | |||
Lingual Lipase | Lingual glands | Triglycerides (fats and oils), other lipids | Fatty acids and diglycerides |
Salivary Amylase | Salivary glands | Polysaccharides (starches) | α-Dextrins, disaccharide (maltose), trisaccharide (maltotriose) |
Gastric juice | |||
Gastric lipase | Stomach chief cells | Triglycerides (fats and oils) | Fatty acids and monoglycerides |
Pepsin* | Proteins | Peptides | |
Brush-border | |||
α-Dextrinase | Small intestine | α-Dextrins | Glucose |
Enteropeptidase | Trypsinogen | Trypsin | |
Lactase | Lactose | Glucose and galactose | |
Maltase | Maltose | Glucose | |
Nucleosidases and phosphatases | Nucleotides | Phosphates, nitrogenous bases, and pentoses | |
Peptidases | Aminopeptidase: amino acid at amino end of peptides Dipeptidase: dipeptides | Aminopeptidase: amino acids and peptides Dipeptidase: amino acids | |
Sucrase | Sucrose | Glucose and fructose | |
Pancreatic juice | |||
Carboxy-peptidase* | Pancreatic acinar cells | Amino acid at carboxyl end of peptides | Amino acids and peptides |
Chymotrypsin* | Proteins | Peptides | |
Elastase* | Proteins | Peptides | |
Nucleases | Ribonuclease: ribonucleic acidDeoxyribonuclease: deoxyribonucleic acid | Nucleotides | |
Pancreatic amylase | Polysaccharides (starches) | α-Dextrins, disaccharide (maltose), trisaccharide (maltotriose) | |
Pancreatic lipase | Triglycerides that have been emulsified by bile salts | Fatty acids and monoglycerides | |
Trypsin* | Proteins | Peptides |
Microscopic Level – Cells
Cells of the Stomach
A single layer of epithelial cells forms the inner most later of the stomach, called the mucosa. The mucosa is marked by depressions called gastric pits (these are lined with epithelial cells). The gastric gland is at the bottom of the pit, which contain multiple cell types: mucous cells, stem cells, parietal cells, chief cells, and G cells (enteroendocrine cells).
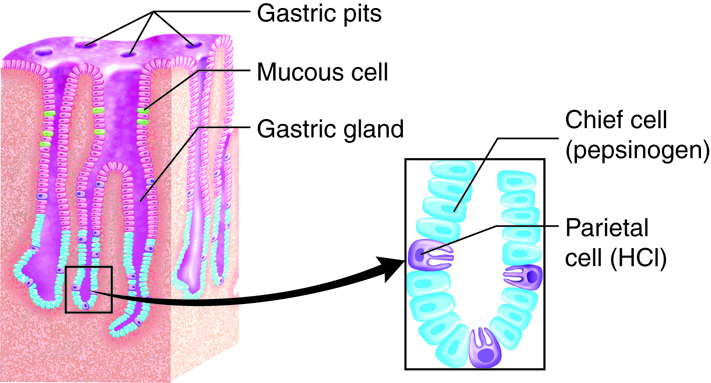
Exocrine Function
Digestion in the stomach requires a combination of acid and pepsin. In the glands of the stomach, chief cells secrete pepsinogen, which is converted to pepsin, as well as gastric lipase, which is used for digestion of fats. Parietal cells secrete hydrochloric acid (HCl) as well as intrinsic factor. The gastric mucous cells associated with the surface and neck of the gastric pit secrete a thick mucus that is more alkaline than the mucus produced by goblet cells found elsewhere in epithelial tissue. This helps provide a protective mucosal barrier from the acid and protein enzymes in the gastric juice.
Endocrine Function
Regulation within the digestive system is tightly controlled by enteroendocrine cells. Collectively, these cells are found in the organs of the digestive system (entero- inside) and release hormones and paracrine signals which regulate production of digestive chemicals and also influence the nervous system for global regulation of digestion. Within the stomach, the enteroendocrine cells are called G-cells.
G-cells secrete the signaling molecules including gastrin. Gastrin signals parietal cells (HCl producing) and chief cells (enzyme producing) to regulate production and alters stomach motility by altering the sphincter function on either side of the stomach. G cells also secrete other paracrines – serotonin, histamine, somatostatin, and other hormones which regulate digestion.
Structure | Action | Outcome |
---|---|---|
Gastric epithelial cells | Structure of the stomach lumen | Maintains structure of the mucosa |
Chief cells | Secrete pepsinogen
Secrete gastric lipase |
Pepsin (activated form) breaks proteins down into peptides
Breaks down triglycerides into fatty acids and monoglycerides |
G cells | Secrete gastrin | Activates secretion of HCl by parietal cells and pepsinogen by chief cells; contracts lower esophageal sphincter, enhances stomach motility, and relaxes pyloric sphincter |
Parietal cells | Secrete HCl
Secrete intrinsic factor |
Kills microbes; denatures proteins, transforms pepsinogen into pepsin
Enables vitamin B12 absorption, which is necessary for red blood cell formation |
Cells of the Small and Large Intestines
Small Intestine Exocrine Function
The small intestine is primarily composed of simple columnar epithelial cells with microvilli facing the lumen for absorption of digested material. Intestinal crypts (also called the crypts of Lieberkühn) are analogous to gastric glands of the stomach and are formed by invaginations of the epithelium. Within the crypts are paneth cells, which secrete multiple defensive proteins including lysozyme definsins and phospholipase to protect the small intestine from pathogens which have survived the stomach compartment. There are also Goblet cells, which secrete mucous for protection from the acids of the stomach and enzymes from accessory organs.
Small Intestine Endocrine Function
The small intestine also has enteroendocrine cells which secrete hormones for regulation of absorption of nutrients. S cells produce secretin to buffer intestinal pH. I cells (also called CCK cells) secrete cholecystokinin, which stimulates the release of digestive enzymes from the pancreas and gall bladder in to the intestine. K cells secrete gastric inhibitory peptide (GIP; also called glucose-dependent insulinotropic peptide), which influences insulin levels.
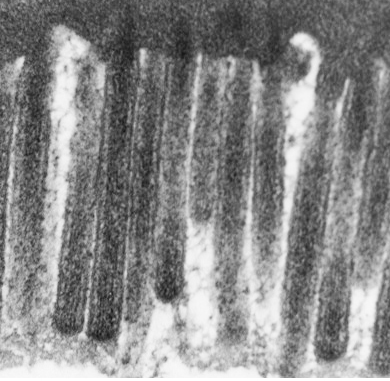
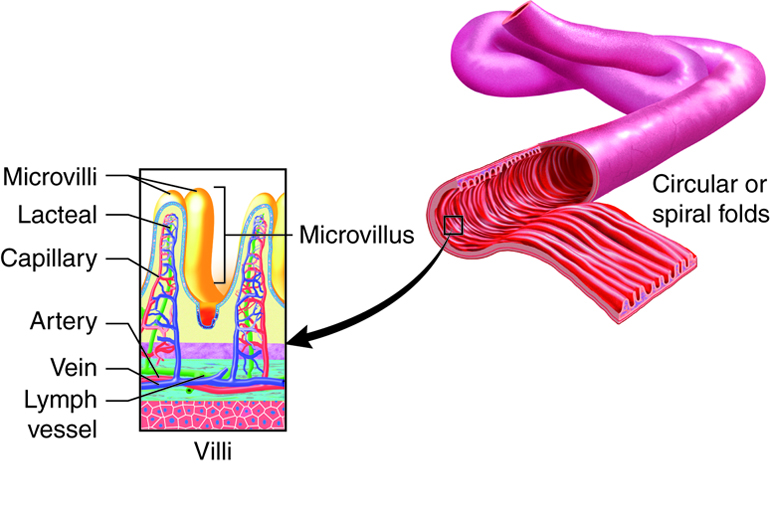
Large Intestine Exocrine Function
Most of the mucosa of the large intestine is composed of simple columnar epithelial cells. An exception is the distal anal canal, which is composed of nonkeratinized stratified squamous epithelial cells. The stratified epithelium is more durable to the abrasion that occurs when feces moves. The large intestine also has crypts, which contain both epithelial cells and goblet cells. Since most digestion and absorption occurs before the large intestine, the only significant secretion is mucus, which lubricates the passage of digestive residue.
Cell Type | Location in the Mucosa | Function |
---|---|---|
Columnar epithelium with microvilli | Lining | Digestion and absorption of nutrients in chyme |
Goblet | Intestinal crypts | Secretion of mucous |
Paneth | Intestinal crypts | Secretion of the bactericidal enzyme lysozyme and other defensive proteins; phagocytosis |
Enteroendocrine | ||
S cells | Intestinal glands | Secretion of the hormone secretin |
I (or CCK) cells | Intestinal glands | Secretion of the hormone cholecystokinin |
K cells | Intestinal glands | Secretion of the hormone gastric inhibitory peptide (GIP) |
Cells of the Liver
Exocrine Function
Hepatocytes are the liver’s main functional cells (hepato – liver, cytes – cells). They play a role in a wide variety of secretory, metabolic, and endocrine functions. Hepatocytes account for around 80 percent of the liver’s volume. Plates of hepatocytes called hepatic laminae (singular lamina) radiate out from the center of hepatic lobules. Hepatocytes continually secrete bile, but production and release accelerate when bile acid levels in the hepatic portal blood (blood coming from the small intestine) increase. This means more bile is secreted as digestion and absorption are proceeding in the small intestine.
Immune Function
The liver also contains star-shaped Kupffer cells that are found in the blood filled spaces (sinusoids) between laminae. These phagocytic cells remove dead or damaged red and white blood cells, as well as bacteria and other foreign material that potentially enter through the GI tract and travel to the liver in the hepatic portal vein.
Cells of the Pancreas
The pancreas is responsible for both endocrine (hormone secretion) and exocrine (enzyme secretion) function. Cells are organized into clusters throughout the pancreas.
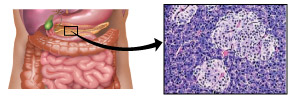
Exocrine Function
The majority of the cells in the pancreas with an exocrine function are acini cells. These epithelial cells were named this because they form clusters around a small central space or lumen that connects to small ducts, looking much like a cluster of grapes (acinus is Latin for berry or grape). Each acinus cell (singular) has a wider basal side, toward the basement membrane, and a narrow apical side toward the lumen, forming a ring or pie-shaped cells. They secrete the various enzymes and bicarbonate ions that make up pancreatic juice into this central duct.
Endocrine Function
The endocrine component of the pancreas consists of islet cells (islets of Langerhans) that create and release important hormones directly into the bloodstream. Two of the main pancreatic hormones are insulin, which acts to lower blood sugar, and glucagon, which acts to raise blood sugar. Maintaining proper blood sugar levels is crucial to the functioning of key organs including the brain, liver, and kidneys. The pancreatic cells responsible for endocrine production are listed below.
Cell type | Percent of islet cells | Endocrine material | Downstream effect |
---|---|---|---|
Alpha cells | 15-20% | Glucagon | Increases blood glucose of glycogen |
Beta cells | 65-80% | Insulin and amylin | Lowers blood glucose |
Delta cells | 3-10% | Somatostatin | Restricts absorption |
PP cells | 3-5% | Pancreatic polypeptide | Regulates pancreatic function |
Tissues
Tissue Layers of the Gastrointestinal Tract
Throughout the gastrointestinal (GI) tract, walls are comprised of the same four fundamental tissue layers. From the lumen of the GI tract, these layers are the mucosa, submucosa, muscularis, and serosa.
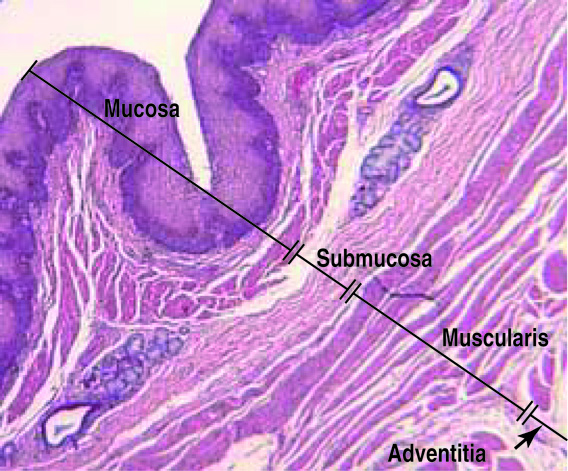
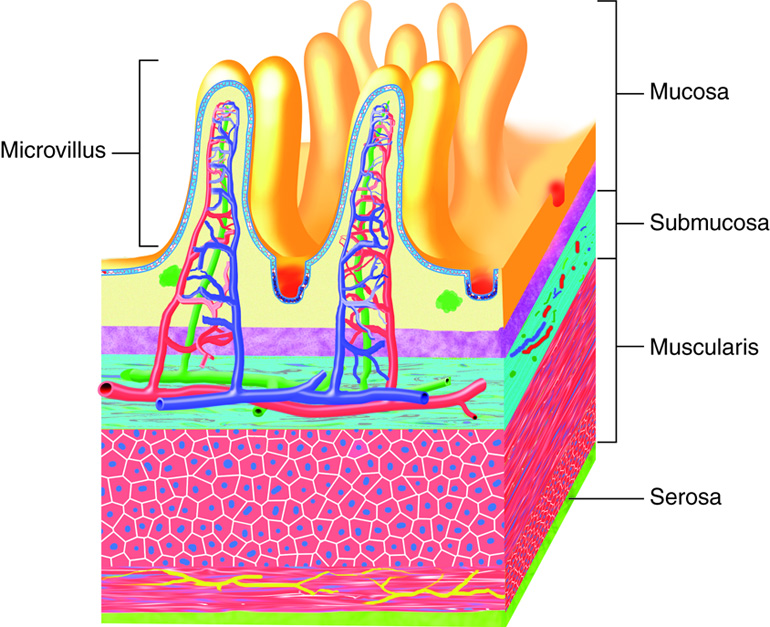
The Mucosa
The mucosa is a mucous membrane that makes up the inner lining of the GI tract. It has three layers: (1) the epithelium, made of closely packed cells without a blood supply or nerves in direct contact with the foodstuffs that enter the GI tract; (2) the lamina propria, a layer of connective tissue that supports the epithelial cells; and (3) a thin smooth muscle layer called the muscularis mucosae. In the mouth, pharynx, esophagus, and distal portion of the anal canal, the epithelium is primarily nonkeratinized stratified squamous epithelium. In the stomach and intestines, it is simple columnar epithelium, whose cells participate in secretion and absorption. Every five to seven days, the harsh chemical and mechanical environment of the GI tract causes epithelial cells to be sloughed off and replaced by new ones. Epithelial cells are interspersed with exocrine cells that secrete mucus and digestive fluid into the lumen (interior space) of the alimentary canal and with enteroendocrine cells that secrete hormones and paracrines. In addition to connective tissue, the lamina propria of the mucosa contains numerous blood and lymphatic vessels, which transport nutrients absorbed into the GI tract to the liver. The lamina propria also contains most of the immune cells that make up the mucosa-associated lymphatic tissue (MALT).
The muscularis mucosae layer is not responsible for movement of material through the GI tract, but it controls the exposed surface area. Small alterations in the many small folds in the mucous membrane of the stomach and small intestine increases the surface area available for digestion and absorption. Of these folds, the rugae in the stomach are temporary structures while the plicae circulares of the small intestine are permanent. When this muscle layer contracts, rugae and plicae circulars are pushed together exposing less SA to the lumen. When it relaxes more of the mucosal surface is revealed and in contact with the digestive products in the lumen.
The Submucosa
The submucosa binds the mucosa to the muscularis externa. It is composed of areolar connective tissue and includes blood and lymphatic vessels (which transport absorbed food molecules) and the submucosal plexus (which is part of nervous system control).
The Muscularis
In the mouth, pharynx, and superior and middle esophagus, the muscularis externa contains skeletal muscle that we use for voluntary swallowing. The external anal sphincter is also made of skeletal muscle, giving us voluntary control of defecation. In the rest of the GI tract, the muscularis externa is smooth muscle, which contracts involuntarily to break down food, mix it with digestive juices, and move it along the GI tract. Complementary muscles in longitudinal (along the length of the tract) and circular layers creates peristalsis – the wave-like muscular movements to move good from the esophagus to the anus.
The Serosa
The serosa is the superficial layer of the intestine that covers the parts of the GI tract that is exposed to the abdominal cavity. This serous membrane is made up of areolar connective tissue and simple squamous epithelium (mesothelium). The esophagus has a single layer of tough connective tissue called the adventitia; it does not have a serosa.
Peritoneum and Peritoneal Folds
The peritoneum, is the largest serous membrane in the body and lines the abdominal cavity. The tissue has several components including the the mesothelium and an underlying supporting areolar connective tissue layer. The connective tissue in turn has two layers: the parietal peritoneum, which lines the abdominal wall, and the visceral peritoneum, which covers some organs and serves as their serosae. The peritoneal cavity is the small space between the parietal and visceral peritoneum that contains the lubricating serous fluid.
The peritoneum includes large folds that bind organs to each other and to the abdominal walls. This keeps the organs in the proper place and suspends them when we are upright. Within these folds are blood vessels, lymphatic vessels, and nerves that innervate the abdominal organs.
Fold | Description | Comment |
---|---|---|
Greater omentum | Drapes over the transverse colon and small intestine | High adipose tissue content vastly expands with weight gain, creating the characteristic “beer belly” |
Falciform ligament | Attaches the liver to the anterior abdominal wall and diaphragm | The liver is the only digestive organ attached to the anterior abdominal wall |
Lesser omentum | Suspends the stomach and duodenum from the liver | Provides a pathway for the blood supply of liver; contains the common bile duct |
Mesentery | Attaches the jejunum and ileum of the small intestine to the posterior abdominal wall | Includes blood and lymphatic vessels, and lymph nodes |
Mesocolon | Attaches the transverse colon and sigmoid colon of the large intestine to the posterior abdominal wall | Along with mesentery, it holds intestines loosely in place, enabling movement with muscle contractions |
Homeostatic Imbalances of the Peritoneum: Peritonitis
Inflammation of the peritoneum is called peritonitis. It can be caused by an injury that penetrates into the abdomen or from an ulcer that perforates the stomach wall, allowing gastric fluids into the peritoneal cavity. The most common cause of peritonitis is a ruptured appendix. The appendix is a terminal part of the cecum (a peritoneal pouch at the beginning of the large intestine), and the function (if any) is still debated. When the appendix bursts open, bacteria-laden feces spurt into the peritoneum. Usually, the peritoneal layers will bind together around the site of inflammation, keeping the infection from spreading, while macrophages move in to dispose of infected tissue. Peritonitis that spreads out into the peritoneal cavity can be life-threatening. The condition is treated by surgically removing the infected tissue and administering high doses of antibiotics. Generally, peritonitis is a concern with any kind of puncture wound to the abdomen.