55 Digestive Structures and Functions
Major Functions of the Digestive System
The digestive system moves water, nutrients and electrolytes from the external environment to the internal environment. Within the digestive system, the gastrointestinal tract is a continuous hollow tube from the mouth to the anus and is technically contiguous with the external environment. This internal space is called a lumen. All digestive system organs play vital roles in breaking down food moving through the gastrointestinal tract into its chemical building blocks, absorbing these building blocks into the blood, and eliminating residual indigestible materials.
Different parts of the digestive system will secrete substances into the digestive tract lumen. These secretions come from both the cells that make up the epithelial lining of the digestive tract and from exocrine accessory organs that have ducts emptying into the tube. These substances will assist with the movement of food from one part of the tract to the next (such as mucus) and with the digestion of the food while it is in the tube (such as enzymes).
In order to absorb digested substances, that material has to be first moved from the lumen, into the apical or tube-side of the epithelial cells lining the digestive tract, then out of the other basal side of those cells, into the body’s interstitial fluid, and into blood or lymph capillaries for transport through the body. This process can happen passively or require ATP cellular energy. You may want to review membrane transport in the cell module. Various chemicals secreted into the lumen can enhance the absorption of some material, such as vitamins and lipids. Anatomical structures that increase the area of the absorptive surface exposed to the digested substances in the tube will also enhance absorption.
There will be another kind of secretion involved in regulating this whole process, including how long food stays in one section of the digestive tract for the most efficient digestion or absorption. These are endocrine secretions from cells that are part of the digestive organs themselves. These hormones are secreted into the blood, not the digestive lumen, and transported by blood to target cells of the digestive system organs. In this way, it helps regulate and coordinate the functions among the different organs. The autonomic nervous system also plays a role in regulating and coordinating the digestive system.
We usually don’t think about the digestive system until something goes wrong with it – when we get indigestion, nausea, or diarrhea. We seldom appreciate that the digestive system is a complex string of organs that make up the body’s engine, turning fuel from the food we eat into energy that keeps us going. Each organ of the digestive system performs specific functions but all these organs work together to digest the foods we eat and absorb the nutrients into our bodies.
Organs of the Digestive System
The digestive system is generally divided into two main categories: organs of the alimentary canal (aliment = “nourish”) and accessory digestive organs. The alimentary canal, also called the gastrointestinal (GI) tract or gut, is a continuous muscular tube that runs from the mouth to the anus. The internal space of this tube is called the lumen. The GI tract is involved with the digestion of food –its breakdown into smaller fragments – and the absorption of digested food fragments from the lument through the alimentary canal wall and into the bloodstream. The accessory digestive organs contribute to secretions to the GI tract, but the food doesn’t pass through these organs.
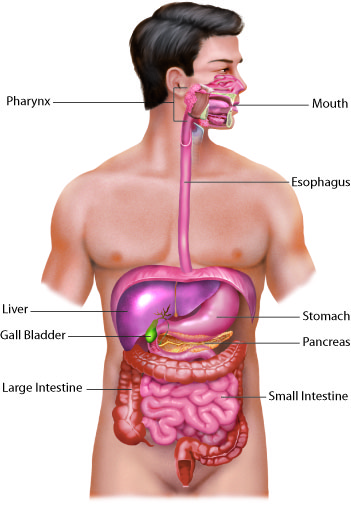
Different digestive system organs are responsible for different digestive processes and functions. These functions include: extracting nutrients from food and removing waste. The processes by which these occur are called ingestion, motility of food through the GI tract lumen, mechanical and chemical digestion of the food, absorption and breakdown of products, defecation to remove residues.
Organ | Major Functions | Other Functions |
---|---|---|
Mouth | Ingests food; Mechanical chewing of food; Salivary amylase begins chemical breakdown of starch; Swallows food and propels it into pharynx | Salivary mucus helps dissolve food; Release of flavors stimulates tastebuds allowing us to appreciate its taste; Saliva moistens food, and tongue helps create a bolus that can be swallowed; Saliva cleans and lubricates the teeth and oral cavity |
Pharynx | Propels bolus from oral cavity to esophagus | Mucus lubricates food passageways |
Esophagus | Peristaltic waves propel food bolus to stomach | Mucus lubricates food passageways |
Stomach | Peristaltic waves combine food with gastric juice and move it into the duodenum; Pepsin begins protein digestion; Absorbs some fat-soluble substances (e.g., alcohol, aspirin) | Hydrochloric acid neutralizes ingested pathogens and stimulates protein-digesting enzymes; Mucus lubricates and protects the stomach; Intrinsic factor allows vitamin B12 to be absorbed in intestines |
Small intestine | Mixes contents with digestive juices for digestion and absorption; Brush-border enzymes digest food; Absorbs breakdown products of carbohydrates, protein, fat, and nucleic acid digestion, along with vitamins, water, and electrolytes | Alkaline mucus helps neutralize acidic chyme from the stomach |
Large intestine | Enteric bacteria digest some food residue and vitamins; Absorbs most residual water, electrolytes, and vitamins produced by enteric bacteria; Propels feces toward rectum; Defecation reflex eliminates feces | Residues are concentrated and temporarily stored prior to defecation; Mucus smoothes passage of feces through colon |
Accessory organs | Liver: produces bile; Gall bladder: stores and concentrates bile; Pancreas: produces enzymes that digests food | Gall bladder releases bile, which emulsifies fat and stimulates the digestion of fat and the absorption of fatty acids, monoglycerides, cholesterol, phospholipids, and fat-soluble vitamins; Bicarbonate-rich pancreatic juice helps neutralize acidic chyme (from the stomach) and provide optimal environment for enzymatic activity |
Movement of Material Through the Digestive System
Both voluntary skeletal muscles and involuntary smooth muscles are involved in propelling food material through the digestive system. Skeletal muscles of the tongue are involved in the voluntary first phase of swallowing, but then it becomes an involuntary reflex with skeletal muscles of the pharynx. The swallowing process is called deglutition.
The smooth muscle of the GI tract is arranged in two layers: longitudinal along the length of the tube and circular around the diameter of the tube. Coordinated contraction and relaxation of these two layers creates a wave-like propulsion of the food called peristalsis. Muscles in the esophagus mechanically transport the food via peristalsis from the mouth to the stomach. An extra smooth muscle layer in the stomach adds a churning movement within the stomach. Peristalsis continues to propel food through the small and large intestines.
Controlling the rate of food moving from one organ of the digestive tract to the next are specialized circularly arranged skeletal and smooth muscles called sphincters. Sphincters are typically contracted and relax only to allow food to move from one organ to the next. The majority of sphincters along the length of the GI tract are involuntary smooth muscle structures. The esophageal sphincter helps separate the esophagus from the stomach to maintain linear movement and protect the esophagus from acidic chemicals of the stomach. The pyloric sphincter separates the stomach from the small intestine; again there is a physical separation for compartmentalization and to maintain directionalized movement.
Material then snakes its way through the small intestine and the iloeocecal sphincter controls movement into the large intestine or colon. The anal sphincter muscle is skeletal muscle controlled consciously to relax during defecation. There is also a hepatopancreatic sphincter that controls the release of accessory secretions from the liver and pancreas into the beginning of the small intestine.
Overview of Digestion
Digestion is accomplished both mechanically and chemically. Mechanical digestion physically breaks food into smaller pieces, increasing surface area for more efficient chemical digestion. Chemical digestion breaks large food macromolecules down into their chemical building blocks, which can then be absorbed through the intestinal wall and into the general circulation.
Food is ingested in the mouth where it begins mechanical digestion from the grinding of the teeth and chemical digestion from enzymes in the saliva. Chemical digestion starts in the saliva and follows into the stomach. In the stomach material is broken down into smaller components by chemically strong acids, enzymes and mechanical churning. However, the small intestine is the site of most chemical digestion, and almost all absorption. Enzymes, bile, bicarbonate and other materials are mixed in from the pancreas and liver to promote chemical digestion and allow enhanced adsorption. Enzymes are responsible for the majority of this chemical digestion. The breakdown of fat also requires emulsification by bile, secreted by the liver and stored in the gall bladder.
Overview of Absorption
The mechanical and chemical digestive processes that begin in the mouth and continue through the small intestine have one endpoint: to convert food into substances that can be absorbed from the lumen by epithelial cells in the lining of the GI tract and then enter blood or lymphatic vessels. Absorbable substances are the monosaccharides, glucose, galactose, and fructose from carbohydrates; single amino acids, dipeptides, and tripeptides from proteins; and monoglycerides, glycerol, and fatty acids from lipids such as triglycerides.
Most nutrients are absorbed into digestive epithelial cells by active transport mechanisms. Nutrients not absorbed through active transport include lipids, lipid-soluble vitamins, and most water-soluble vitamins. With the help of bile salts, the breakdown products of lipids are transported into intestinal cells in structures called micelles. These absorbed fats then aggregate into chylomicrons for transport.
These substances absorbed into the epithelial cells of the digestive tract are then absorbed into either the circulatory or lymphatic capillaries for transport where needed by body cells for metabolism. Absorption from the GI lumen is enhanced by increasing the surface area. Most absorption occurs in the small intestine, the longest organ of the GI tract. Several anatomical structures at the organ, tissue, and cell level also improve nutrient, water and electrolyte absorption.
Plicae circulares
On the organ scale, the inside lining of the small intestine lumen is not smooth. Plicae circulares, are permanent ridges or folds forming successive rings along the length of the small intestine. These folds increase the surface area of the lining exposed to contents in the lumen for a given length of small intestine, which allows increased absorption.
Villi
At the microscopic tissue level, there are finger-like extensions of the epithelial and connective tissue called villi. The villi increase the surface area of the epithelial tissue exposed to contents moving through the lumen. This allows more superficial epithelial cells to absorb within a square millimeter of the lining than if this same millimeter surface were flat. Within each villi, deep to the epithelial basement membrane, the connective tissue supports the villi structure as it extends into the lumen. Capillary networks that pick up and transport absorbed substances through the body extend from the deeper connective tissue into each villus (singular of villi). These include blood capillaries and a central specialized lymph capillary, called a lacteal, for lipid absorption.
Microvilli
At the cellular level, the apical surface of each epithelial cell has cell membrane extensions into the lumen called microvilli. These cells are collectively known as brush border cells because their combined microvilli along the surface of each villus looks like the surface of a brush under the microscope. Because of the microvilli, each epithelial cell has a longer cell membrane exposed to the lumen contents than it would if its apical surface were smooth, improving cellular absorption. Embedded in the cell’s folded plasma membrane are different cell membrane proteins: transporters and enzymes. The transporters absorb by active transport, but the brush border enzymes are catalysts that complete the digestion of only partially digested proteins and carbohydrates at the cell surface, so they can pass through the adjacent transporters.
Surface Area
Explanation of increased surface of the small intestine due to the characteristics of its structures.
Mouth
The cheeks, tongue, hard palate, and soft palate frame the mouth, also called the oral cavity or buccal cavity. The mouth is involved in both mechanical and chemical digestion. Mechanical digestion consists of mastication (chewing), in which the tongue manipulates food, the teeth grind it, and saliva mixes with it. Mastication turns food into an easy-to-swallow bolus and breaks the food into smaller pieces so that there is more contact area for digestive enzymes.
The cheeks make up the oral cavity’s lateral walls. The outer covering of the cheeks is the skin, and the inner covering is the mucous membrane. This membrane is made up of nonkeratinized stratified squamous epithelium; the multiple layers are resistant to abrasion. Between the skin and mucous membranes are connective tissue, fat tissue and buccinator muscles.
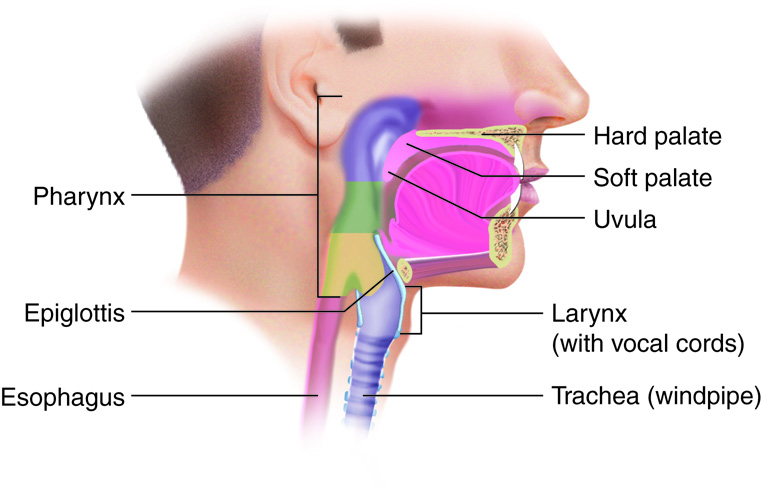
The lips, also called labia (“fleshy borders”), encircle the opening of the mouth. Their outer covering is skin, and their inner lining is mucous membrane. Between these two layers is the orbicularis oris muscle. The labial frenulum is a midline fold of mucous membrane that attaches the inner surface of each lip to the gum. When we chew food, the buccinator muscles in the cheeks and the orbicularis oris muscle in the lips contract, which keeps food between the lower and upper teeth. These muscles also play a role in speech.
The area between the lips (or cheeks) and teeth is called the oral vestibule. The oral cavity is the area than runs between the gums and teeth and the entrance to the throat (oropharynx), also called the fauces (“passages”). If you puff out your cheeks, you can increase the size of the oral vestibule but not the oral cavity.
The septum that separates the oral cavity and nasal cavity is called the palate. Anatomically, a septum (plural, septa) is a wall within a single organ or cavity that separates the space into distinct sides. For example, the nasal septum divides the nostrils. This is separating two distinct cavities.
The palate, which forms the roof of the mouth, is what allows us to breathe while chewing food. The anterior part of the roof of the mouth is called the hard palate. It is created by the maxillae and palatine bones and is covered by mucous membrane. The hard palate makes up the bony wall between the oral and nasal cavities. The posterior part of the roof of the mouth, the soft palate, is also lined with mucous membrane. This arch-shaped muscular structure forms a dividing wall between the oropharynx and nasopharynx.
The uvula (Latin for ‘little grape’) is a cone-shaped muscular process that hangs from the end of the soft palate. It plays a role in speech and articulation of words. It also plays a small role in preventing foods and liquids from entering the nasal cavity. Two muscular folds on each side of the base of the uvula extend down the lateral sides of the soft palate. The anterior fold is called the palatoglossal arch, which terminates next to the base of the tongue. The posterior fold, the palatopharyngeal arch, lies at the interface with the pharynx. Between these two arches on the lateral wall are the palatine tonsils. The lingual tonsils are located at the base of the tongue.
There are also many small, intrinsic salivary glands within the mucous membrane of the mouth and tongue. Their secretion is independent of the presence of food. These glands either open directly into the oral cavity or indirectly through short ducts. Small amounts of saliva are secreted by the labial glands in the lips, the buccal glands in the cheeks, the palatal glands in the palate, and the lingual glands in the tongue.
Tongue
The tongue is composed of skeletal muscle covered with a surface of nonkeratinized stratified squamous epithelium and a mucous membrane covering. The tongue and its associated muscles make up the floor of the oral cavity. A median septum extends the entire length of the tongue, dividing it into symmetrical halves. Inferiorly, the tongue is attached to the mandible, styloid process of the temporal bone, and hyoid bone.
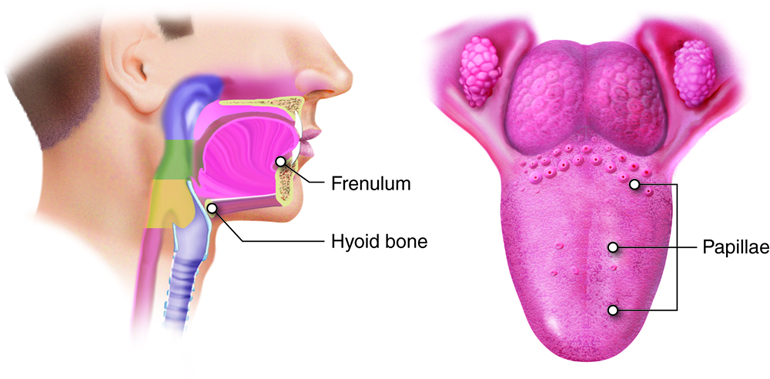
Each half of the tongue has the same number and type of intrinsic and extrinsic muscles. As you learned in your studies of the muscular system, extrinsic muscles of the tongue are the hyoglossus, genioglossus, styloglossus and palatoglossus muscles. These muscles originate outside the tongue and insert into connective tissues within the tongue. They move the tongue from side to side and in and out, performing three important digestive functions: (1) moving food for optimal chewing, (2) shaping food into a bolus (rounded mass), (3) pushing food toward the back of the mouth to be swallowed and (4) elevates the posterior part of the tongue to assist in swallowing. Conscious control of the tongue also aids in speaking and other non-digestive processes.
The top and sides of the tongue are studded with papillae (“nipple-shaped”) extensions of lamina propria layer of mucosa, which are enshrouded in stratified squamous epithelium. Most of these papillae contain taste buds; those without taste buds have touch receptors to give a sense of food’s texture. The latter increase the amount of friction between the tongue and food, which helps the tongue move food around in the mouth. Lingual glands, are found on the anterior, inferior surface of the tongue, in the lamina propria of the tongue secrete mucus and a watery serous fluid that contains the enzyme lingual lipase, which begins the digestion of triglycerides.
Teeth
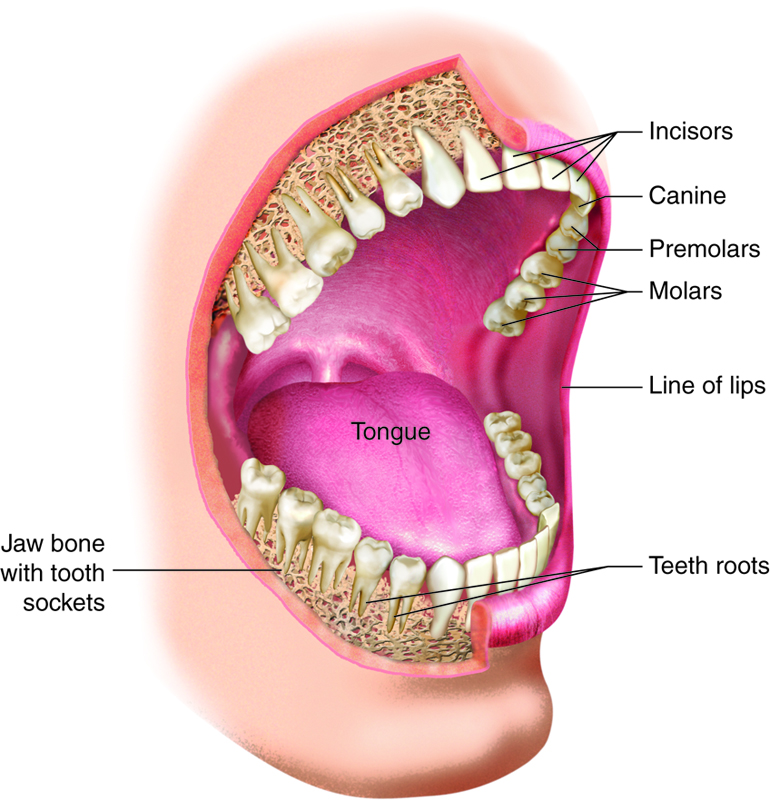
The teeth, or dentes, are secured in sockets of the alveolar processes of the maxillae and mandible. Gingivae (gums; singular gingiva) cover the alveolar processes. Lining the sockets is the periodontal ligament, a dense fibrous connective tissue that secures the teeth in place. The teeth are covered by enamel, which is the hardest substance in the body. Enamel helps prevent teeth from being worn down when we chew, and it helps keep out acids that could easily dissolve the interior of a tooth.
Humans have two sets of teeth (dentitions). The 20 deciduous teeth, or baby teeth, first appear at about 6 months of age. Between ages 6 and 12 years, these teeth are replaced by the 32 permanent teeth. Closest to the midline are the two incisors, chisel-shaped teeth we use for cutting into food. The cuspids (canines) are next to the incisors. A cuspid’s pointed edge (cusp) tears and shreds food. Posteriorly, the next teeth are the two premolars (bicuspids) followed by up to three molars. Premolars and molars have broader, flatter surfaces, which we use to crush and grind food.
Structure | Action | Outcome |
---|---|---|
Lips and cheeks | Confine food between teeth | Foods are chewed evenly during mastication |
Salivary glands | Secrete saliva | Moistens and lubricates the lining of mouth and pharynx
Moistens, softens, and dissolves food so we can taste Cleans mouth and teeth Salivary amylase breaks down starch |
Tongue – Extrinsic muscles | Tongue moves sideways, in and out, and elevates posteriorly | Maneuvers food for chewing
Shapes food into bolus Maneuvers food for deglutition (swallowing) |
Tongue – Intrinsic muscles | Tongue changes shape | Maneuvers food for deglutition (swallowing) |
Tongue – Taste buds | Sense taste and presence of food in mouth | Nerve impulses from taste buds are conducted to salivatory nuclei in brain stem and then to salivary glands, stimulating saliva secretion |
Lingual glands | Secrete lingual lipase | Breaks down triglycerides into fatty acids and diglycerides |
Teeth | Cut, tear, and crush food | Breaks down solid food into smaller particles for deglutition (swallowing) |
Pharynx
The pharynx (throat) is a funnel-shaped tube that runs from the choanae (internal nostrils) to the esophagus posteriorly and to the larynx anteriorly. The pharynx has three subdivisions: the nasopharynx, the oropharynx, and the laryngopharynx. The nasopharynx acts as a passageway for air during ventilation, while the other two subdivisions participate in both ventilation and digestion. Superiorly, the oropharynx is connected with the nasopharynx, inferiorly with the larynx and laryngopharynx, and anteriorly with the mouth.
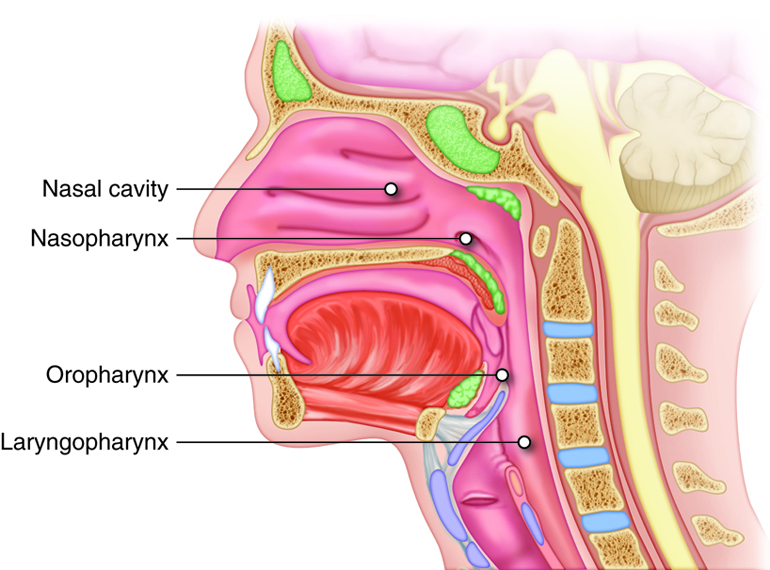
Histologically, the wall of the pharynx is similar to that of the oral cavity. The mucosa includes a epithelium with mucous-producing glands. The nasopharynx is lined with pseudostratified columnar epithelium; the oropharynx and laryngopharynx are lined with stratified squamous. There are two skeletal muscle layers in the external muscle layer of the pharyngeal wall. Cells in the inner layer extend longitudinally. In the outer layer, the superior, middle, and inferior pharyngeal constrictor muscles are stacked, one inside the other, like flowerpots. Contraction of these muscles propels food toward the esophagus.
When swallowed food enters the oropharynx and laryngopharynx, it provokes contractions of the pharyngeal constrictor muscles that help push food into the esophagus. Usually during swallowing, the soft palate moves back to close off the nasopharynx, while the trachea (“windpipe”) moves up under the epiglottis to cover the glottis (the opening to the larynx or voice box); this effectively blocks off air passages. But we all know what it feels like to have food “go down the wrong tube,” which means it either goes into the nasal cavities or the trachea. When food enters the trachea, our reaction is to cough, which usually forces the food up and out of the trachea and back into the pharynx.
Esophagus
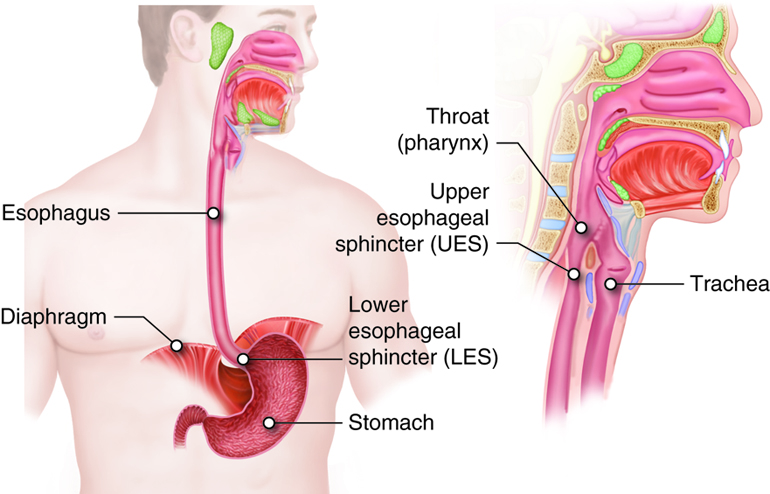
The esophagus is a collapsible tube located posterior to the trachea. This muscular tube is about 10 inches long. The esophagus runs a mainly straight route through the mediastinum of the thorax. To enter the abdomen, the esophagus penetrates the diaphragm through an opening called the esophageal hiatus. At the cardiac orifice, the esophagus joins with the stomach. Surrounding this orifice is the lower esophageal sphincter (also called the gastroesophageal or cardiac sphincter). Remember that sphincters are circular muscles that surround tubes and serve as valves, closing the tube when the sphincters contract and opening it when they relax. The lower esophageal sphincter relaxes to let food pass into the stomach, then contracts to prevent stomach contents from backing up into the esophagus. Surrounding this sphincter is the muscular diaphragm, which helps close off the sphincter when no food is being swallowed. When the lower esophageal sphincter does not completely close, some of the stomach contents can reflux (i.e., back up into the esophagus), causing heartburn or gastroesophageal reflux disease (GERD).
The esophageal mucosa is made up of nonkeratinized stratified squamous epithelium, lamina propria, and a muscularis mucosa. The mucosa near the stomach also includes mucous glands. The stratified squamous epithelium protects against abrasion and erosion from food particles. The superficial layer of the esophagus is called the adventitia, not the serosa. In contrast to the stomach and intestines, the areolar connective tissue of the adventitia is not covered by mesothelium.
The esophagus secretes mucus that lubricates food, and it propels food into the stomach. Chemical digestion that began in the mouth continues in the esophagus, but there aren’t any new digestive enzymes secreted. The upper esophageal sphincter controls the movement of food from the pharynx into the esophagus. The lower esophageal sphincter controls the movement of food from the esophagus into the stomach. Rhythmic waves of peristalsis begin in the esophagus and then continue in the rest of the digestive tract organs. The feeling of having a lump in your throat when you are nervous is caused by peristalsis that occurs when there is no food in the esophagus.
Action | Outcome |
---|---|
Upper esophageal sphincter relaxation | Allows bolus to move from the laryngopharynx to the esophagus |
Peristalsis | Propels bolus down the esophagus |
Lower esophageal sphincter relaxation | Allows bolus from the esophagus to enter the stomach |
Mucus secretion | Lubricates the esophagus, allowing easy passage of bolus |
Deglutition
Deglutition is another word for swallowing – the movement of food from the mouth and into the stomach. The entire process takes about four to eight seconds for solid or semisolid food and about one second for very soft food and liquids. Deglutition involves the mouth, pharynx, and esophagus. It is facilitated by the secretion of mucus and saliva. There are three stages in deglutition: the voluntary phase, the pharyngeal phase, and the esophageal phase.
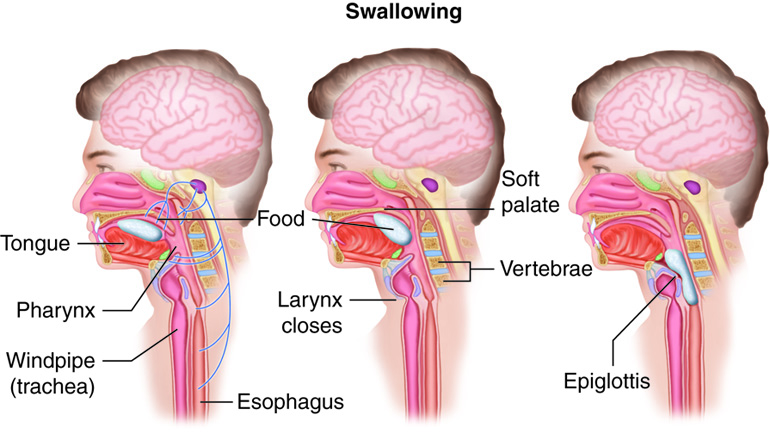
The voluntary phase of deglutition is also called the oral phase or buccal phase. In this phase, swallowing is set in motion when the tongue moves upward and backward against the palate, pushing the bolus to the back of the oral cavity and into the oropharynx. At this point, the two involuntary phases of swallowing begin.
In the pharyngeal phase, stimulation of receptors in the oropharynx sends impulses to the deglutition center (a collection of neurons that controls swallowing) in the medulla oblongata. Impulses are then sent back to the uvula and soft palate, provoking them to move upward to close off the nasopharynx. Sequential contractions of the pharyngeal constrictor muscles move the bolus through the oropharynx and laryngopharynx. Relaxation of the upper esophageal sphincter then allows food to enter the esophagus.
The entry of food into the esophagus marks the beginning of the esophageal phase of deglutition. Peristalsis propels the bolus through the esophagus and toward the stomach. The circular muscle layer of the muscularis immediately superior to the bolus contracts, pinching the esophageal wall, forcing the bolus forward. At the same time, the longitudinal muscle layer of the muscularis inferior to the bolus also contracts, shortening this inferior area and pushing out its walls to receive the bolus. Waves of contractions keep moving the food toward the stomach. When the bolus nears the stomach, relaxation of the lower esophageal sphincter allows the bolus to pass into the stomach. During the esophageal phase, esophageal glands secrete mucus that lubricates the bolus and minimizes friction. Peristalsis is completely involuntary even though there is skeletal muscle in the first 2/3 of the esophagus.
Stomach
The stomach is an expansion of the GI tract that lies below the esophagus. It is a very mobile structure between its relatively fixed upper and lower ends. The stomach is found between the esophagus to the first part of the small intestine (the duodenum). The stomach’s position and size are always changing. It is about as big as a large sausage when empty, and stretches to hold 1 liter of food when full; the stomach has a final capacity of 2-3 liters. One of the digestive functions of the stomach is to serve as a temporary “holding chamber”. This is an important step in digestion, because we can eat a meal far more quickly than it can be digested and absorbed in the small intestine. So the stomach pushes only a small amount of food into the small intestine at a time.
The stomach plays several important roles in chemical digestion, including the continued digestion of starch and the initial digestion of proteins and triglycerides. The stomach is also where the semisolid bolus is converted to a liquid (chyme) and where some ingested substances are absorbed.
Stomach Anatomy
There are four main regions in the stomach: the cardiac, fundus, body, and pylorus. The cardiac (cardia region) is a small area surrounding the cardiac orifice through which food from the esophagus enters the stomach. Lying beneath the diaphragm, superior and to the left of the cardiac, is the dome-shaped fundus, which functions as a temporary storage center for food. Inferior to the fundus is the body, the large central part of the stomach. The funnel-shaped pylorus connects the stomach to the duodenum. The pyloric antrum is the wider, more superior portion of the pylorus that connects to the body of the stomach. The pyloric antrum narrows into a region called the pyloric canal, which leads into the duodenum. The pylorus communicates with the duodenum via the smooth muscle pyloric sphincter. This sphincter controls stomach emptying. In the absence of food, the stomach deflates inward and its mucosa and submucosa fall into large folds called rugae.
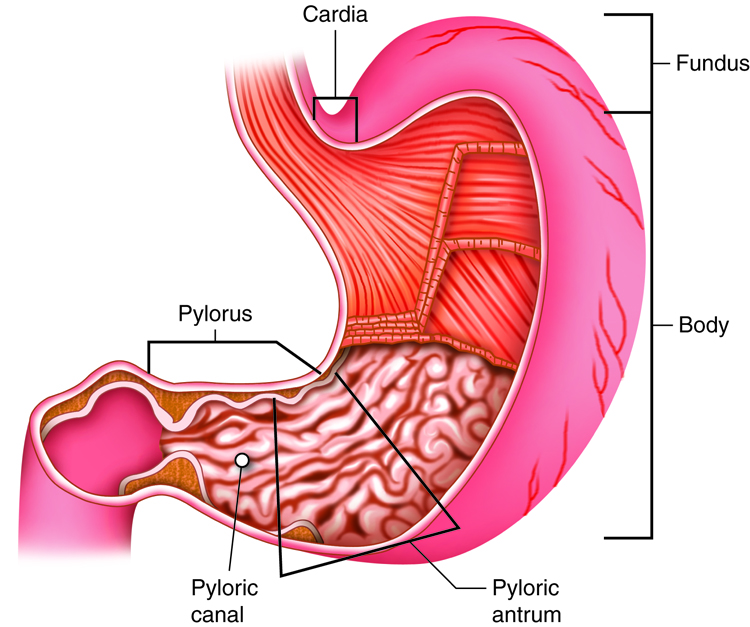
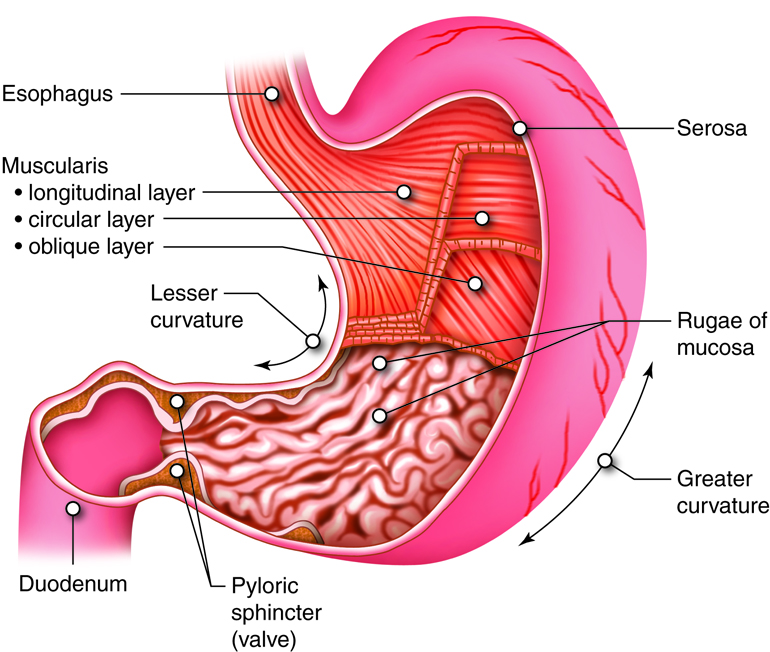
The convex lateral surface of the stomach is called the greater curvature; the concave medial border is the lesser curvature. Two fatty mesenteries (peritoneal folds) called omenta hold the stomach in place. The lesser omentum extends from the liver to the lesser curvature. The greater omentum runs from the greater curvature to the posterior abdominal wall.
Adaptations of the Stomach Wall
The wall of the stomach is made of the same four tissue layers as most of the rest of the GI tract, but with adaptations to the mucosa and muscularis externa for the unique functions of this organ. In addition to the typical circular and longitudinal smooth muscle layers, the muscularis externa has an inner oblique smooth muscle layer that runs diagonally around the organ. As a result, in addition to moving food through the GI tract, the stomach can more vigorously churn and mix food, mechanically breaking it down into smaller particles.
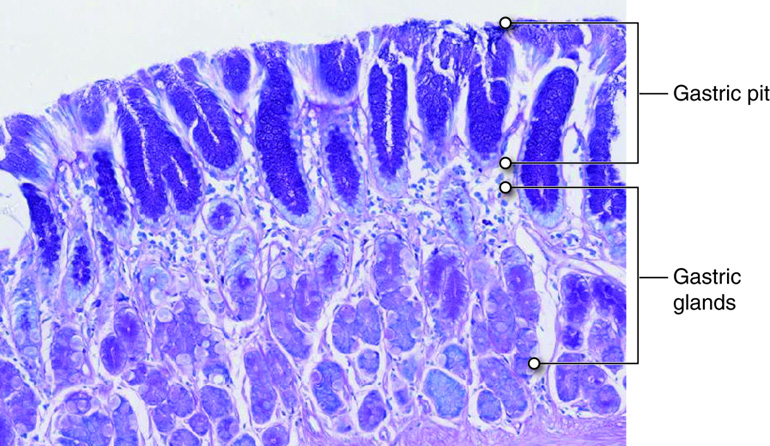
Millions of deep gastric pits in the epithelium lead into gastric glands, which secrete gastric juice. The walls of the gastric pits are made up primarily of epithelial cells. The gastric glands are made up of different types of cells. Glands of the cardia and pylorus are composed primarily of mucus-secreting cells. Cells that make up the pyloric antrum secrete mucus and a number of hormones, including gastrin. The much larger glands of the fundus and body of the stomach, the site of most chemical digestion, produce most of the gastric secretions. These glands are made up of a variety of secretory cells, including mucous neck, parietal, chief, and enteroendocrine cells (G-cells).
Structure | Action | Outcome |
---|---|---|
Muscularis Externa | Mixing waves
Peristalsis |
Forms chyme by liquefying food and mixing it with gastric juice
Propels chyme through pyloric sphincter |
Pyloric sphincter | Controls passage of chyme from the stomach to the duodenum | Delivers chyme to the intestine at a rate optimal for digestion and absorption
Prevents chyme from flowing back to stomach from duodenum |
Chief cells | Secrete pepsinogen
Secrete gastric lipase |
Pepsin (activated form) breaks proteins down into peptides
Breaks down triglycerides into fatty acids and monoglycerides |
G cells | Secrete gastrin | Activates secretion of HCl by parietal cells and pepsinogen by chief cells; contracts lower esophageal sphincter, enhances stomach motility, and relaxes pyloric sphincter |
Parietal cells | Secrete HCl
Secrete intrinsic factor |
Kills food microbes; denatures proteins, transforms pepsinogen into pepsin
Enables vitamin B12 absorption for red blood cell formation |
Surface mucous cells and mucous neck cells | Secrete mucus
Absorption |
Protects stomach wall from digestive processes
Allows limited amount of water, ions, short-chain fatty acids, and certain drugs to enter bloodstream |
Digestive Functions of the Stomach
The stomach participates in virtually all digestive activities with the exception of ingestion and defecation. In addition to mechanical and chemical digestion, the stomach absorbs some fat-soluble substances, including alcohol and aspirin.
Mechanical Digestion
A few minutes after food enters the stomach, mixing waves begin at intervals of about 15 to 25 seconds. Mixing waves are a gentle type of peristalsis that soften and moisten food. They also combine food with gastric juice and create chyme. Initial gentle mixing waves are followed by more intense waves that break down food into smaller pieces and further mix with digestive juice, starting at the body of the stomach and increasing in force as they reach the pylorus.
The pylorus, which holds around 30 mL (1 ounce) of chyme, acts as a filter, permitting only liquids and small food particles to pass through the mostly, but not fully, closed pyloric sphincter. When food enters the pylorus, periodic mixing waves force about 3 mL of chyme through the pyloric sphincter and into the duodenum, a process called gastric emptying. The rest of the chyme is pushed back into the body of the stomach, where it continues mixing. This process is repeated when the next mixing waves force more chyme into the duodenum. These forward and backward movements accomplish most of the mixing in the stomach.
Gastric emptying is regulated by both the stomach and the duodenum. The presence of chyme in the duodenum activates receptors that inhibit gastric emptying. This prevents additional chyme from being released by the stomach before the duodenum is ready to process it.
Chemical Digestion
Some foods sit in the fundus of the stomach for an hour or so before they are mixed with gastric juice. During this time, the digestive activity of salivary amylase continues. Mixing waves soon take over however, combining chyme with acidic gastric juice, which inactivates salivary amylase and activates lingual and gastric lipase. Lingual lipase then starts breaking down triglycerides into fatty acids and diglycerides.
The digestion of protein begins in the stomach, primarily by pepsin. During infancy, gastric glands also produce rennin, an enzyme that helps digest milk protein. Lingual lipase secreted by the salivary glands may also participate in triglyceride digestion in the stomach. Parietal cells secrete hydrogen ions and chloride ions into the stomach lumen, effectively forming HCl, which denatures dietary proteins.
The contents of the stomach are completely emptied into the duodenum within two to four hours after you eat a meal. Carbohydrate-rich foods are the quickest to leave the stomach. High-protein foods exit a little more slowly. Fatty meals with high triglyceride content remain in the stomach the longest. Enzymes in the small intestine digest fats slowly. So when the duodenum is processing fatty chyme, food can stay in the stomach for six hours or longer.
Small Intestine
The small intestine is a convoluted tube that begins just distal to the pyloric sphincter of the stomach and then loops through the central and inferior region of the abdomen before ending at the ileocecal valve, where it merges with the large intestine. The small intestine is the primary digestive organ in the body. Not only is it the part of the GI tract where digestion is completed, it is also where the majority of absorption occurs.
Although the small intestine is the longest part of the GI tract, its diameter is about half that of the large intestine, averaging a little over one inch (2.5 cm). When we are alive, the small intestine is more than 3 meters (10 feet) long – the size of a one-story building. Its length provides expansive surface area necessary for digestion and absorption. However, circular folds, villi, and microvilli add even more surface area. With loss of muscle tone after death, the folds of the small intestine relax, extending it to about 20 feet in length.
Small Intestine Anatomy
The duodenum precedes the jejunum and ileum and is the shortest part of the small intestine; it is less than 1 foot of the 10 foot intestine (30 cm of the 3 m). The duodenum receives the stomach contents, pancreatic juice and bile. Chemical digestion continues in the duodenum. The jejunum is the next portion (3.5 – 5.5 ft or 110-170 cm) of the small intestine and is responsible for the absorption of a majority of nutrients. In the last section of the small intestine, the ileum, vitamin B12 and bile salts are absorbed as well as materials not absorbed by the jejunum.
The term mucosa or mucous membrane always refers to the combination of the epithelium plus the lamina propria. The lamina propria layer of the small intestinal mucosa is composed of areolar connective tissue and quite a bit of mucosa-associated lymphoid tissue. Most solitary lymphatic nodules are located in the distal portion of the ileum. The blood supply which runs through the lamina propria and drains the villus is part of the the hepatic portal systems and not general systemic circulaions
In the ileum, the lamina propria also has aggregated lymphatic follicles (groups of lymphatic nodules) called Peyer’s patches. There are more Peyer’s patches toward the end of the small intestine, possibly because of the high amounts of bacteria in that area must be prevented from entering the bloodstream. The submucosa of the duodenum is the only site of the complex mucus-secreting duodenal (Brunner’s) glands, which produce a bicarbonate-rich alkaline mucus that helps buffer the acidic chyme coming in from the stomach.
Like in the rest of the GI tract, the muscularis externa layer is made of two layers of smooth muscle – an outer, thinner layer of longitudinal fibers and an inner, thicker layer of circular fibers. The serosa completely enshrouds the small intestine, with the exception of a large region of the duodenum.
Absorptive Function of the Small Intestine
Each day, the alimentary canal processes up to 10 liters of food, liquids, and GI secretions; of which, only about one liter enters the large intestine. Almost all ingested food and drink, 80 percent of electrolytes, and most of the water are absorbed in the small intestine. The entire small intestine is involved in absorption, but most absorption occurs before chyme reaches the ileum. Absorption in the ileum primarily involves the recycling of bile salts . The absorptive capacity of the alimentary canal is amazing. By the time chyme passes from the ileum into the large intestine, it is essentially indigestible food residue (mainly plant fibers like cellulose) some water, and millions of bacteria.
The absorption of most nutrients through the mucosa of the intestinal villi occurs via active transport mechanisms that are driven directly or indirectly by metabolic energy. These nutrients enter the capillary blood in the villus and travel to the liver via the hepatic portal vein . An exception is certain lipids, which undergo passive absorption via diffusion and then enter the modified lymph duct in the villus (called a lacteal ), to be transported to the blood in lymphatic fluid. Substances cannot be absorbed between the epithelial cells of the intestinal mucosa, because these cells connect with tight junctions. This is why substances can only enter blood capillaries by passing through the epithelial cells and into the interstitial fluid.
Carbohydrate Absorption
All carbohydrates are absorbed in the form of monosaccharides. The small intestine is highly efficient at this, absorbing monosaccharides at an estimated rate of 120 grams per hour. All normally digested dietary carbohydrates are absorbed. However, indigestible fibers including cellulose, glucose polymers made by plants which is not broken down in humans, are eliminated in feces. The monosaccharides glucose and galactose – the products of the breakdown of starch and disaccharides – are transported into the epithelial cells by common protein carriers via secondary active transport, which requires ATP). They leave these cells via facilitated diffusion and enter the capillaries through intercellular clefts. The monosaccharide fructose (which is in fruit) is absorbed and transported by facilitated diffusion alone. Monosaccharides combine with the transport proteins, which lie very near the disaccharide splitting enzymes on the microvilli, immediately after disaccharides are broken down.
Protein Absorption
Active transport mechanisms, primarily in the duodenum and jejunum, absorb most proteins as their breakdown products, amino acids. Almost all (95 to 98 percent) protein is digested and absorbed in the small intestine. The type of carrier that transports an amino acid varies. Most carriers are linked to the active transport of sodium. Short chains of two amino acids (dipeptides) or three amino acids (tripeptides) are also transported actively. But after they enter the absorptive epithelial cells, they are broken down to their amino acids before leaving the cell and entering the capillary blood via diffusion.
Lipid Absorption
Despite being hydrophobic, the small size of short-chain fatty acids enables them to be absorbed in intestinal chyme, enter absorptive cells via simple diffusion, and then take the same path as monosaccharides and amino acids into the blood capillary of a villus. The large and hydrophobic long-chain fatty acids and monoglycerides are not so easily suspended in the watery intestinal chyme. However, bile salts and the phospholipid lecithin (also found in bile) help make them more soluble by surrounding them and creating tiny spheres called micelles. Micelles are macromolecular structures made up of aggregates of fatty elements and bile salts that create a polar (hydrophilic) end that faces the water and a nonpolar (hydrophobic) core. The core also includes cholesterol molecules and fat-soluble vitamins. Micelles can easily squeeze between microvilli and get very near the luminal cell surface. At this point, lipid substances exit the micelle and are absorbed via simple diffusion. Without their “vehicles” (i.e., the micelles), lipids would sit on the surface of chyme, like oil on water, and would never come in contact with the absorptive surfaces of the epithelial cells. About 95 percent of lipids are absorbed in the small intestine. Bile salts not only speed up lipid digestion, they are also essential to the absorption of the end products of lipid digestion.
The free fatty acids and monoglycerides that enter the epithelial cells are reincorporated into triglycerides, which are then mixed with phospholipids and cholesterol and surrounded with a protein coat, creating chylomicrons. Chylomicrons are milky-white droplets of water-soluble lipoprotein that are processed by the Golgi apparatus for release from the cell. Chylomicrons are too big to pass through the basement membranes of blood capillaries. Instead, they enter the much larger pores of lacteals. This means that most fats are first transported in the lymphatic vessels and do not enter the venous blood until they reach the thoracic duct in the neck region. In the bloodstream, the enzyme lipoprotein lipase breaks down the triglycerides of the chylomicrons into free fatty acids and glycerol. These two breakdown products can then pass through capillary walls to become energy used by our cells or to be stored in adipose tissue as fats. Liver cells combine the remaining chylomicron material with proteins, forming “new” lipoproteins that transport cholesterol in the blood.
Nucleic Acid Absorption
The products of nucleic acid digestion – pentose sugars, nitrogenous bases, and phosphate ions –are transported across the epithelium by special carriers in the villus epithelium via active transport.
Electrolyte Absorption
The electrolytes absorbed by the small intestine are from both GI secretions and ingested foods and liquids. Recall that electrolytes are compounds that separate into ions in water. Most ions are absorbed throughout the entire small intestine. Exceptions are iron and calcium, which are primarily absorbed in the duodenum.
In the small intestine, sodium ion absorption is linked via co-transport mechanisms to the absorption of glucose and amino acids. A sodium-potassium pump moves sodium ions from the basolateral side of small intestine epithelial cells into the bloodstream they enter. Chloride ions are also absorbed via active transport. Changing osmotic gradients passively move potassium ions across the intestinal mucosa via facilitated diffusion (or via leaky tight junctions).
In general, all nutrients that enter the intestine are absorbed, whether we need them or not. Iron and calcium are exceptions; they are absorbed in amounts that fulfill the needs of our body. The iron ion needed for the production of hemoglobin is absorbed into mucosal cells via active transport. Once inside mucosal cells, ionic iron binds to the protein ferritin, creating iron-ferritin complexes that act as intracellular storage facilities for iron. When the body has enough iron, most of the stored iron is lost when worn-out epithelial cells slough off. When the body needs iron because, for example, it is lost during acute or chronic bleeding, there is increased uptake of iron from the intestine and accelerated release of iron into the blood. Because women experience significant iron loss during menstruation, they have around four times as many iron transport proteins in their intestinal epithelial cells as men.
Blood levels of ionic calcium (Ca2+) determine the absorption of calcium. The active form of vitamin D, under the control of parathyroid hormone, provides local regulation of this process in the intestines. When blood levels of ionic calcium drop, parathyroid hormone (PTH) secreted by the parathyroid glands stimulates the release of calcium ions from bone matrix and increases the reabsorption of calcium by the kidneys. PTH also provokes the activation of vitamin D by the kidneys, which triggers greater calcium ion absorption in the small intestine.
Vitamin Absorption
Vitamins in our food are absorbed in the small intestine. Some of the vitamin K and B vitamins created by enteric bacteria are absorbed in the large intestine. Fat-soluble vitamins (A, D, E, and K) are absorbed along with dietary lipids in micelles via simple diffusion. In fact, you need some fat in your diet in order to be able to absorb fat-soluble vitamins. Some of the B vitamins are not actually found in our food but are made by gut bacteria. Most water-soluble vitamins are absorbed by simple diffusion , including most B vitamins and vitamin C. An exception is vitamin B12, which is a very large molecule. Intrinsic factor secreted in the stomach binds to vitamin B12, creating a combination that can bind to mucosal receptors in the terminal ileum, launching its active uptake by endocytosis.
Water Absorption
Each day, about nine liters (9.8 quarts) of fluid enter the small intestine; about 2.3 liters are ingested, and the rest is from GI secretions. About 95 percent of water is absorbed in the small intestine. The absorption of water in the alimentary canal occurs via osmosis. Around 300 to 400 mL of water is absorbed per hour. Water can move easily in both directions across the intestinal mucosa. The active transport of solutes into mucosal cells establishes a concentration gradient that results in water moving into the cells via the process of osmosis. As water enters the cells, it concentrates the solutes left in the lumen, enhancing the absorption of those molecules absorbed by passive diffusion. This means that the absorption of water is essentially linked to the absorption of solutes, which, in turn, determines the absorptive rate of those salts and sugars that are absorbed via diffusion. The water that moves into the mucosal cells is followed by these substances along their concentration gradients.
Large Intestine
The large intestine is the terminal part of the gastrointestinal tract. The primary digestive function of this organ is to finish absorption, produce some vitamins, form feces, resorb water and eliminate feces from the body.
The large intestine runs from the cecum, where it attches to the ileum, to the anus. It borders the small intestine on three sides. Despite its being around half as long as the small intestine – 4.9 feet versus 10 feet (1.5 – 3 meters) – it is called the large intestine because it is more than twice the diameter of the small intestine, 2.5 inches versus one inch (6 cm versus 2.5 cm). The large intestine is tethered to the posterior abdominal wall by the mesocolon, a double layer of peritoneal membrane.
The large intestine is subdivided into four main regions: the cecum, the colon, the rectum, and the anus. The ileocecal valve, located at the opening between the ileum in the small intestine and the large intestine, controls the flow of chyme from the small to the large intestine.
Large Intestine Anatomical Structures
Like the small intestine, the mucosa of the large intestine has intestinal glands that contain both absorptive and goblet cells. However, there are several notable differences between the walls of the large and small intestines. For example, other than the anal canal, the mucosa of the colon is simple columnar epithelium. In addition, the wall of the large intestine has no circular folds, no villi, and essentially no enzyme-secreting cells. This is because most nutrients are already absorbed before chyme enters the large intestine. The large intestinal wall does have thicker mucosa and deeper – and more abundant – glands that contain a vast number of goblet cells. These goblet cells secrete mucus that eases the movement of feces and protects the intestine from the effects of the acids and gases produced by enteric bacteria.
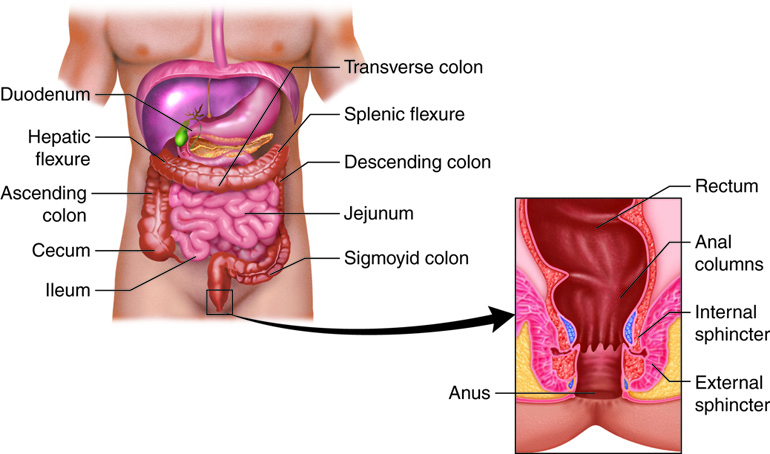
The stratified squamous epithelial mucosa of the anal canal joins with the skin around the anus. This mucosa varies considerably from that of the rest of the colon to accommodate the increased abrasion in this region. The anal canal’s mucous membrane is organized in longitudinal folds called anal columns that house a grid of veins. Depressions between the anal columns, called anal sinuses, secrete mucus when they are crowded by feces. This facilitates defecation. The pectinate line is a horizontal, jagged band that runs alongside the inferior margins of the anal sinuses. The mucosa superior to this line is fairly insensitive to pain, while the area inferior to this line is very pain-sensitive. The difference in pain response is due to the fact that the superior region is innervated by visceral sensory fibers, and the inferior region is innervated by somatic sensory fibers. There are two superficial venous plexuses in the anal canal – one with the anus and the other with the anal columns. Inflammation and distension of these (hemorrhoidal) veins causes hemorrhoids, an itchy condition caused by the swelling of these vessels.
Three features are unique to the large intestine: teniae coli, haustra, and epiploic appendages. The teniae coli are three bands of smooth muscle that make up the longitudinal muscle layer of the muscularis externa of the large intestine, except at its terminal end in the rectum. Tonic contractions of the teniae coli bunch up the colon into a succession of pouches called haustra, which are responsible for the wrinkled appearance of the colon. Attached to the teniae coli are small, fat-filled sacs of visceral peritoneum called epiploic appendages (omental appendices). These fatty pouches of peritoneum found in the serosa from the transverse colon through the sigmoid colon.
Although the rectum and anal canal have no teniae coli or haustra, they do have well-developed layers of muscularis externa muscle that create the strong contractions needed for defecation.
Large Intestine Gross Anatomy
The first part of the large intestine is the cecum, a small sac-like region that is suspended inferior to the ileocecal valve. This cecum is about 2.4 inches long. The appendix or vermiform appendix (vermiform= “worm-shaped”, and appendix = “appendage”) is a winding, coiled tube that attaches to the cecum. This 2-7 cm (~3 inch) long appendix contains lymphoid tissue and plays an important role in immunity. Nevertheless, its twisted anatomy provides a haven for the accumulation and multiplication of enteric bacteria. The mesoappendix, the mesentery of the appendix, tethers it to the inferior part of the mesentery of the ileum.
The end of the cecum joins with the colon, a long tube with several distinct areas. The ascending colon runs up the right side of the abdomen. At the inferior surface of the liver, it takes a right-angle turn, forming the right colic (hepatic) flexure and becoming the transverse colon. The transverse colon runs across to the left side of the abdomen. It then bends sharply at a point immediately anterior to the spleen, forming the left colic (splenic) flexure. As the descending colon, it runs down the left side of the posterior abdominal wall. After entering the pelvis inferiorly, it becomes the s-shaped sigmoid colon, which extends medially to the midline. Most of the colon is between the peritoneal membrane and the body wall, except for the intraperitoneal transverse and sigmoid colons, which are tethered to the posterior abdominal wall by mesocolons.
The sigmoid colon joins the rectum in the pelvis, near the third sacral vertebra. The rectum is the final 8 inches (20 cm) of the alimentary canal. It extends anterior to the sacrum and coccyx. Even though rectum is Latin for “straight,” this structure has three lateral bends that create a trio of internal transverse folds called the rectal valves. These valves allow passing of gas (flatus) while preventing the simultaneous passage of feces.
The last part of the large intestine is the anal canal, which is located in the perineum, completely outside the abdominopelvic cavity. This 1 inch (3 cm) long structure opens to the exterior of the body at the anus. The anal canal includes two sphincters. The involuntary internal anal sphincter is made of smooth muscle; the voluntary external anal sphincter is skeletal muscle. Except when defecating, these two sphincters are usually closed.
Bacterial Flora in the Large Intestine
There are many species of bacteria that populate the large intestines. They digest food products for which we do not have enzymes (such as plant materials) and we absorb some of the nutrient products. They can also produce some vitamins like vitamin K and B vitamins. A byproduct of bacterial fermentation (digestion) is gas (flatus).
Although the bacteria provide additional nutrients from the food we ingest, they can also infect us. Because of the large and diverse bacterial population that resides in the large intestine, the large intestine also contains plentiful lymphatic tissue to protect us from potentially harmful effects of resident bacteria.
Most bacteria that migrate to the cecum from the small intestine have already been killed by lysozyme and other antibacterial molecules, HCl, and protein-digesting enzymes. Those that are still living, along with bacteria that come into the alimentary canal through the anus, are referred to as the large intestine’s (enteric) bacterial flora. The more than 700 species of bacterial flora in the colon participate in chemical digestion and absorption. The large intestine is also home to a number of viruses and protozoans, at least 20 of which are known pathogens.
Most enteric bacteria are nonpathogenic commensals (also called symbiotic, wityh benefit to both organisms) that cause no harm as long as they stay in the gut lumen. A refined system prevents these bacteria from crossing the mucosal barrier. First, certain bacterial components activate the release of chemicals by the mucosa’s epithelial cells, which recruit immune cells, especially dendritic cells, into the mucosa. Dendritic cells open the tight junctions between epithelial cells and extend probes into the lumen to evaluate the microbial antigens. The dendritic cells then travel to neighboring lymphoid follicles in the mucosa and hand over the antigens to T cells. This triggers an IgA antibody-mediated response in the lumen that blocks the commensals from infiltrating the mucosa and setting off a far greater, widespread systematic reaction.
Digestive Function of the Large Intestine
The major digestive role of the large intestine involves propulsion–pushing fecal matter toward the anus and then out of the body. Chyme, which stays in the large intestine for 12 to 24 hours, contains few nutrients. Enteric bacteria are responsible for a small amount of digestion. The bacterial flora create vitamins required for normal metabolism, such as certain B vitamins and vitamin K. Most of the remaining water and some electrolytes (especially sodium and chloride) are recycled.
Structure | Action | Outcome |
---|---|---|
Lumen | Bacterial degradation of chyme components | Breaks down undigested proteins, carbohydrates, and amino acids into substances that can be absorbed and detoxified by the liver or eliminated in feces; synthesizes vitamin K and some B vitamins |
Mucosa | Mucus secretion
Absorption |
Lubricates colon; protects mucosa
Absorbs water, solidifies feces, and helps maintain water balance in body; absorbed solutes include ions and certain vitamins |
Muscularis externa | Haustral churning
Peristalsis Defecation reflex |
Muscle contractions move contents between haustrums
Contractions of circular and longitudinal muscles propel contents along length of colon Propels contents into sigmoid colon and rectum Contractions in sigmoid colon and rectum eliminate feces |
Examples: Ileostomy
It may surprise you that the large intestine can be completely removed without significantly affecting digestive functioning. For example, if colon cancer necessitates the removal of the large intestine, an ileostomy can be performed, in which the terminal ileum is moved out to the abdominal wall. A sac is then attached to the abdominal wall to collect eliminated food residues.
Mechanical Digestion
Mechanical digestion in the large intestine begins when chyme moves from the ileum into the cecum, an activity regulated by the ileocecal valve. This sphincter is usually partially closed, allowing slow movement of chyme into the large intestine. Right after we eat, peristalsis in the ileum is escalated by the gastroileal reflex, which forces whatever chyme is in the ileum into the cecum. The activity of the hormone gastrin also relaxes the ileocecal valve. When the cecum is distended with chyme, contractions of the ileocecal sphincter strengthen. Once chyme enters the cecum, colon movements begin. As food residues pass the ileocecal valve, they fill the cecum and gather in the ascending colon.
The presence of food residue in the colon stimulates slow-moving haustral contractions (haustral churning). These sluggish segmentations, primarily in the transverse and descending colons, occur about every 30 minutes and last about one minute. When a haustra is distended with chyme, its muscle contracts, pushing the residue into the next haustra. The movements also mix the food residue, which helps the large intestine absorb water.
The large intestine also has peristaltic movements, but they are slower than in more proximal portions of the alimentary canal, at a rate of from three to 12 contractions per minute. The third type of movement in the large intestine is called mass movements (mass peristalsis). These drawn-out, slow-moving, but strong peristaltic waves start around the middle of the transverse colon and quickly force the contents of the colon into the rectum. Mass movements usually occur three or four times per day, either while we eat or immediately afterward. Distension in the stomach and the breakdown products of digestion in the small intestine provoke the gastrocolic or duogenocolic reflex, which increases motility, including mass movements, in the colon. Fiber in the diet both softens the stool and increases the power of colonic contractions, optimizing the activities of the colon.
Chemical Digestion
The glands of the large intestine secrete only mucus; they do not secrete digestive enzymes. So chemical digestion in the large intestine occurs only through the activity of the bacteria in the lumen of the colon. Bacteria ferment residual carbohydrates in the chyme and discharge the hydrogen sulfide, carbon dioxide, and methane gases that help create flatus (gas) in the colon, (flatulence refers to excessive flatus). Some of these gases, including dimethyl sulfide, have foul odors. Each day, about 500 mL of flatus is produced in the colon. Much more is produced when we eat some fiber-rich foods such as beans.
Absorption and Excretion Functions of the Large Intestine
After chyme has been in the large intestine for three to 10 hours, water absorption changes it into the solid or semisolid substance called feces (“stool”). Feces is composed of undigested food residues (dietary fiber), unabsorbed digested substances, millions of bacteria, sloughed-off epithelial cells from the GI mucosa, inorganic salts, mucus, fat and just enough water to let it pass smoothly out of the body. Of every 500 mL (17 ounces) of food residue that enters the cecum each day, about 150 mL (5 ounces) becomes feces.
Although the small intestine absorbs around 90 percent of the water that enters the GI tract, the water absorbed in the large intestine is important in maintaining the body’s water balance. For every 0.5 to 1.0 liter (16 to 32 ounces) of water that goes into the large intestine, only around 100 to 200 mL (3 to 7 ounces) escapes being absorbed by osmosis.
The process of defecation begins when mass movements force feces from the sigmoid colon into the rectum, stretching the rectal wall and provoking the defecation reflex , which eliminates feces from the rectum. This parasympathetic reflex is mediated by the spinal cord. It contracts the sigmoid colon and rectum, relaxes the internal anal sphincter, and initially contracts the external anal sphincter. The presence of feces in the anal canal sends a signal to the brain, which gives us the choice of voluntarily opening the external anal sphincter (defecating) or keeping it temporarily closed. When we decide to delay defecation, it takes a few seconds for the reflex contractions to stop and the rectal walls to relax. The next mass movement will trigger another defecation reflex and another until we defecate.
Feces is eliminated during defecation through contraction of the rectal muscles. We help this process by a voluntary procedure called the Valsalva maneuver, in which we increase intra-abdominal pressure by contracting our diaphragm and abdominal wall muscles and closing our glottis. Along with learned control of the external anal sphincter, these actions must be learned, which is why infants and young children wear diapers.
Diet, health, exercise and stress determine the frequency of bowel movements. The number of bowel movements varies greatly between individuals, ranging from two or three per day to three or four per week.
Accessory Organs
Salivary Glands
Lying outside the oral mucosa are the three pairs of major salivary glands, which secrete the majority of saliva into ducts that open into the mouth. The parotid glands lie between the skin and the masseter muscle near the ears. They secrete saliva into the mouth through the parotid duct, which is located near the second upper molar tooth. The submandibular glands, which are in the floor of the mouth, secrete saliva into the mouth through the submandibular ducts near the lower central incisor. The sublingual glands, which lie below the tongue, use the lesser sublingual ducts to secrete saliva into the oral cavity.
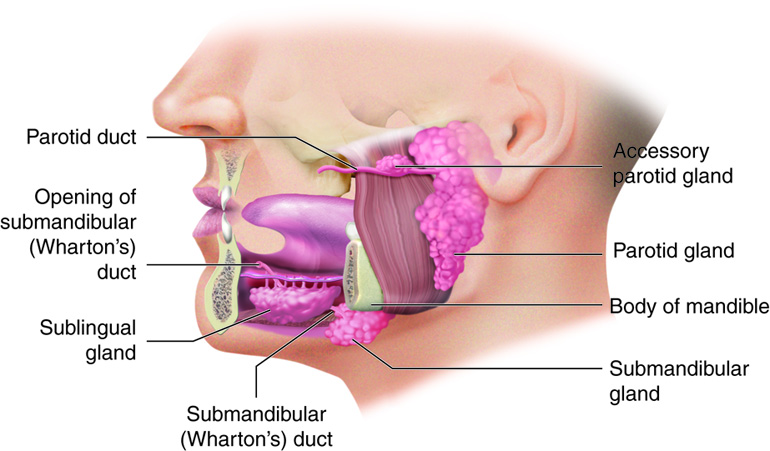
Different salivary glands secrete unique formulations of saliva according to their cellular makeup. For example, the parotid glands secrete a watery solution that contains salivary amylase. The submandibular glands have cells similar to those of the parotid glands, along with some mucous-secreting cells. So saliva secreted by the submandibular glands also contains amylase but in a liquid thickened with mucus. The sublingual glands contain mostly mucous cells, and they secrete the thickest saliva with the least amount of salivary amylase.
Example: Homeostatic Imbalances of the Parotid Glands: Mumps
Infections of the nasal passages and pharynx can attack any salivary glands. The parotid glands are the usual site of infection with the virus that causes mumps (Paramyxovirus). Mumps manifest with enlargement and inflammation of the parotid glands, causing the characteristic swelling between the ears and the jaw. Symptoms include fever and throat pain, which can be severe when swallowing acidic substances such as orange juice.
The autonomic nervous system regulates salivation (the secretion of saliva). An average of 1 to 1.6 quarts (0.9-1.5 liters) of saliva is secreted each day. In the absence of food, parasympathetic stimulation keeps saliva flowing continuously to moisten mucous membranes and lubricate tongue and lip movements when we talk; swallowed saliva moistens the esophagus. Most saliva is reabsorbed, preventing fluid loss. During times of stress, sympathetic stimulation takes over, reducing serous salivation and causing dry mouth. When you are dehydrated, salivation stops to conserve water, causing the mouth dryness that helps stimulate feelings of thirst. Having a drink restores body water homeostasis and moistens the mouth.
Salivation from the major salivary glands is stimulated by both the taste and sensation of food. Food contains chemicals that stimulate taste bud receptors on the tongue, which send impulses to the superior and inferior salivatory nuclei in the brain stem. These two nuclei then send back parasympathetic impulses through fibers in the facial and glossopharyngeal nerves to stimulate salivation. Even after we swallow food, salivation is increased to cleanse the mouth and to dilute and neutralize any irritating chemical remnants — such as that hot sauce in your burrito. Salivation can also be stimulated by simply thinking about, seeing, or smelling food.
Pancreas
Chemical digestion in the small intestine relies on the activities of three accessory digestive organs: the pancreas, liver and gall bladder. The pancreas produces pancreatic juice, which contains digestive enzymes and bicarbonate, and delivers it to the duodenum.
The digestive role of the liver is to produce bile and export it to the duodenum. The gall bladder primarily stores and releases bile.
The soft, tadpole-shaped pancreas is a gland that is about 5-6 inches (13-15 cm) long and 1 inch (2.5 cm) thick. It lies posterior to the greater curvature of the stomach. The pancreas regions are described as the head, body, and tail. The head is next to the duodenum. The body lies behind the stomach. The tail is in contact with the spleen.
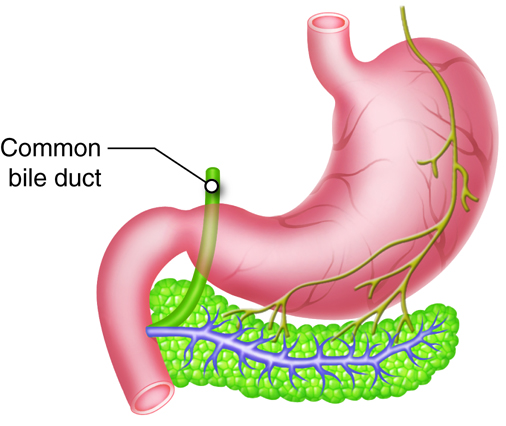
Pancreatic Ducts
The pancreas is important in digestion, because it produces pancreatic juice, a combination of fluid and digestive enzymes. Exocrine cells release this juice into small ducts that eventually unite to create two larger ducts that deliver pancreatic juice to the small intestine. The larger of these two ducts is the centrally located main pancreatic duct (duct of Wirsung). In most individuals, this duct fuses with the common bile duct from the liver and gall bladder before entering the duodenum via the hepatopancreatic ampulla (ampulla of Vater), a dilated common duct. This ampulla opens on the major duodenal papilla, an elevation of the duodenal mucosa, which is situated approximately 4 inches (10 cm) inferior to the pyloric sphincter of the stomach. The smooth muscle hepatopancreatic sphincter controls the flow of pancreatic juice and bile into the small intestine. The second large pancreatic duct, the accessory duct (duct of Santorini) runs from the pancreas directly into the duodenum, approximately 1 inch (2.5 cm) above the hepatopancreatic ampulla.
Pancreatic Islets
In the pancreas, small clusters of glandular epithelial (secretory) cells surround the ducts. The exocrine structures of the pancreas contains 99 percent of the clusters that are called acini (singular – acinus). Cells of the acini secrete pancreatic juice. The endocrine part of the pancreas is made up of the remaining 1 percent of clusters, which are called pancreatic islets (islets of Langerhans). These cells produce the hormones pancreatic polypeptide, insulin, glucagon, and somatostatin. Insulin and glucagon are important in carbohydrate metabolism.
Pancreatic Acini and Exocrine Digestive Function
Regulation of pancreatic secretion is the job of both hormones and the parasympathetic nervous system. The hormone secretin, whose secretion is stimulated by the presence of HCl in the intestine, provokes cells of the pancreatic duct to release bicarbonate-rich pancreatic juice. The presence of fats (and some proteins) in the intestine stimulates the secretion of the hormone cholecystokinin (CCK), which then stimulates the release enzyme-rich pancreatic juice and enhances the activity of secretin. As a result, much more bicarbonate-rich juice is released in the presence of both hormones. Parasympathetic regulation occurs mainly during the cephalic and gastric phases of gastric secretion, when vagal stimulation prompts the secretion of pancreatic juice.
Liver
The liver is the largest gland in the body, as well as one of the most important organs. It plays a number of major roles in metabolism and regulation. In an adult, the reddish-brown liver weighs about 3 pounds (1.4 kg) This wedge-shaped organ lies inferior to the diaphragm in the right upper quadrant of the abdominal cavity. The liver is almost completely surrounded by the rib cage, which offers it some protection.
Anatomical Structures of the Liver
The liver has different levels of structure and function, and different models have been used to describe the organizational and functional relationships between hepatocytes, bile canaliculi, and hepatic sinusoids. The hepatic lobule was considered the functional unit of the liver in years past. In this model, each hexagonal (six-sided) hepatic lobule has a central vein. Radiating from this vein are rows of hepatocytes and hepatic sinusoids.
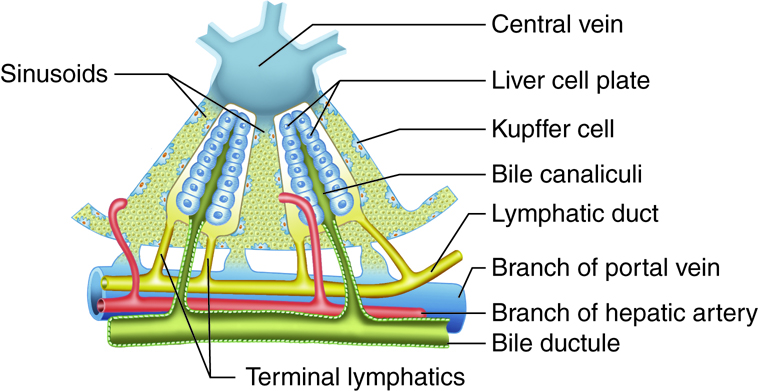
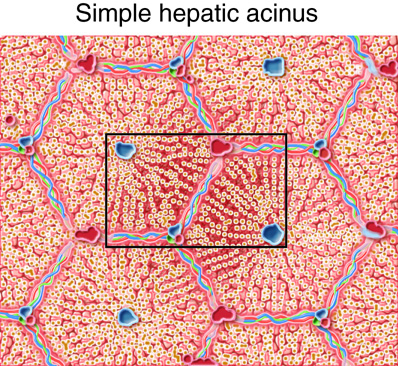
The hepatic acinus has become the preferred model of the metabolic functional unit of the liver in recent years. Each primarily oval hepatic acinus includes parts of two adjacent hepatic lobules. Branches of the portal triad that run along the hepatic lobule border delineate the short axis of the hepatic acinus. The long axis is created by two imaginary curved lines that connect the two central veins nearest the short axis. In the hepatic acinus, hepatocytes are organized in three zones around the short axis. Zone 1 cells, which are nearest the portal triad branches, are the first to receive oxygen, nutrients, and toxic substances from incoming blood. After a meal, zone 1 cells are the first to absorb glucose and store it as glycogen; during fasting, they are the first to break down glycogen to glucose. If circulation is disrupted, zone 1 cells are the last to die and the first to regenerate. Zone 3 cells, which are farthest away from the portal triad branches, die first if circulation is disrupted and are the last to regenerate. They are also the first to demonstrate the accumulation of fat. Predictably, the structural and functional qualities of zone 2 cells lie between those of zone 1 and 3 cells.
The popularity of the hepatic acinus model is based on its reasonable description of glycogen storage and release patterns, as well as the degeneration, regeneration, and toxic effects in the three zones according to their proximity to portal triad branches.
Gross Anatomy of the Liver
The liver is divided into two primary lobes, a large right lobe and a smaller left lobe. Separating the right and left lobes anteriorly is a mesentery called the falciform ligament, which also helps suspend the liver in the abdominal cavity. A number of anatomists believe that the right lobe also includes an inferior quadrate lobe and a posterior caudate lobe, which are defined by internal features. Ligaments connect the liver to other visceral organs, and the visceral peritoneum enshrouds almost the entire liver, except for the part that abuts the diaphragm. The lesser omentum tethers the liver to the lesser curvature of the stomach. The porta hepatis (“gateway to the liver”) is where the hepatic artery and hepatic portal vein enter the liver on its inferior surface. These two vessels, along with the common hepatic duct, run through the lesser omentum on the way to their destinations.
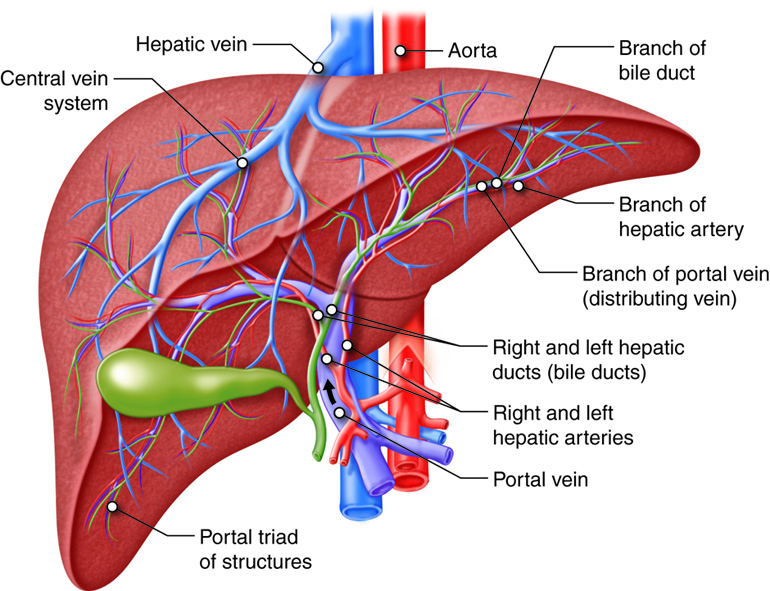
The hepatic artery delivers oxygenated blood from the heart to the liver. The portal system in general has a direct connection between two organs bypassing the systemic circulation. The hepatic portal vein delivers deoxygenated blood containing newly absorbed nutrients, drugs, and possible bacteria from the alimentary canal. Branches of both vessels bring blood into liver sinusoids, where hepatocytes take up most nutrients and some of the toxins. The hepatocytes secrete substances, including nutrients needed by other cells, back into the blood, which drains into the central vein and then the hepatic vein. In this hepatic portal circulation, blood from the GI tract passes through the liver before entering the systemic circulation. This is why the liver is a common site for the metastasis of cancer that originates in the alimentary canal.
Liver Ducts
The liver has three main components: hepatocytes, bile canaliculi, and hepatic sinusoids. Between adjacent hepatocytes, grooves in the cell membranes provide room for bile canaliculi (“small canals”). These small ducts accumulate the bile produced by hepatocytes. From here, bile flows first into bile ductules and then into bile ducts. The bile ducts unite and form the larger right and left hepatic ducts, which themselves merge and exit the liver as the common hepatic duct. This duct then joins with the cystic duct from the gall bladder, forming the common bile duct through which bile flows into the small intestine. Bile production increases when fat-rich chyme stimulates the release of secretin from intestinal cells. Between meals, when most absorption is complete, the hepatopancreatic sphincter prevents bile flow into the duodenum, and bile backs up into the gall bladder, where it is stored until needed.
Liver Circulation
Hepatic sinusoids are specialized blood vessels, more like dilated channels, located between rows of hepatocytes. Branches of the hepatic artery deliver oxygenated blood to hepatic sinusoids; while branches of the hepatic portal vein deliver nutrient-laden blood. The hepatic sinusoids combine and send blood into a central vein. Blood then flows into the hepatic veins, which drain into the inferior vena cava. This means that blood and bile flow in opposite directions. The hepatic sinusoids also contain the immune cells called Kupffer cells. A distinctive arrangement in the liver called the portal triad includes three basic structures: a bile duct, a hepatic artery branch, and a hepatic portal vein branch.
Liver Ligaments
The liver is covered with a visceral peritoneum. These ligaments are useful anatomic positions but serve little physiological purpose aside from attaching the liver to the body wall and other structures. The ligamentum teres (round ligament) runs along the inferior border of the falciform ligament. Tapered extensions of the parietal peritoneum, called the right and left coronary ligaments, hang the liver from the diaphragm.
Gall Bladder
The gall bladder stores and concentrates the bile produced by the liver. The green colored gall bladder lies in a shallow area on the posterior surface of the right lobe of the liver. This pear-shaped sac, which is about 3-4 inches (7-10 cm) long, usually suspends from the anterior inferior margin of the liver. The gall bladder is divided into three regions. The wide fundus runs inferiorly, past the inferior border of the liver. The central portion of the gall bladder is called the body, and the tapered portion the neck. These two areas project superiorly.
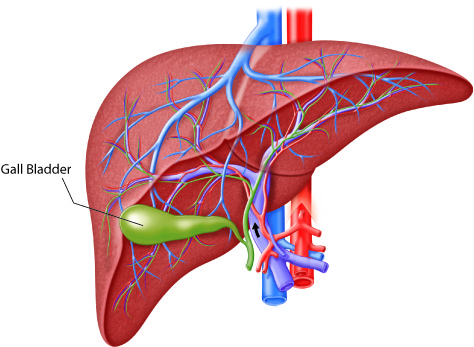
The simple columnar epithelium of the gall bladder mucosa is organized in rugae, similar to those of the stomach. There is no submucosa in the gall bladder wall. The wall’s middle, muscular coat is made of smooth muscle fibers. When these fibers contract, the gall bladder’s contents are released into the cystic duct. Visceral peritoneum forms the outer coat of the gall bladder.
Movement of Bile
The ducts and mechanisms involved in the movement of bile.
Review of Accessory Structures
The primary digestive role of the liver is to produce bile and deliver it to the duodenum. Bile salts separate large fat globules that enter the small intestine into millions of small fatty droplets with large surface areas that are amenable to the activity of fat-digesting enzymes.
The pancreas produces pancreatic juice, whose high alkalinity helps neutralize acidic chyme in the duodenum, while providing the ideal environment for the actions of intestinal and pancreatic enzymes.
The digestive function of the gall bladder is to store and concentrate the bile produced by the liver and then release it when it is needed in the small intestine. The gall bladder’s mucosa absorbs water and ions from bile, concentrating it by as much as twenty-fold.
Organ | Function in the Digestive Process | Outcome |
---|---|---|
Liver | Produces bile, which includes bile salts | Bile salts emulsify lipids for and digestion and absorption |
Pancreas | Produces pancreatic juice and sends it to the duodenum via the pancreatic duct | Pancreatic juice contains a variety of digestive enzymes |
Gall bladder | Stores and concentrates bile | Sends bile to the duodenum via the common bile duct |
Salivary Glands | Produce saliva | Begin to break down carbohydrates and to cleanse the mouth |