48 Lymphatic Levels of Organization
Your exploration of immunity in the following modules will extend from the simplest to the most complex. Although the levels of organization are introduced in modules, the functional interconnections between the organizational levels will build throughout the development of modules within this unit.
- Molecular level of organization (includes 4 general categories of molecules):
- Three main types of antimicrobial substances (interferon, complement, iron-binding transferrins)
- Substances that contribute to aspects of inflammation (histamine, kinins, prostaglandins, leukotrienes, and complement)
- Molecules present on pathogens and infected self-cells (pathogen-associated molecular patterns, antigens and major histocompatibility complex) that are recognized by receptors (pattern recognition receptors, B-cell receptor, T-cell receptor) on immune cells
- Small protein hormones, called cytokines that stimulate or inhibit normal cell functions such differentiation and growth
- Cell level – Neutrophils, Eosinophils, Basophils, Monocytes, Macrophages, Dendritic cells, Natural killer cells and B and T Lymphocytes
- Tissue level – lymph and lymph nodules such as mucosa-associated lymph tissue and tonsils
- Organ level – lymph capillaries and vessels, primary lymph organs such as bone and thymus, secondary lymph organs such as lymph nodes, and spleen
- System level – all components that function together to drain excess interstitial fluid and carry out immune responses
Molecular Level of Organization
Types of Antimicrobial Proteins
Blood and interstitial fluids contain three main types of antimicrobial proteins that interfere with microbial growth: 1) interferons, 2) complement, and 3) iron-binding proteins.
Virus-infected cells produce proteins called interferons (IFNs). IFNs diffuse through the interstitial fluid to uninfected neighboring cells. These proteins then cause the uninfected cells to synthesize antiviral proteins that interfere with viral replication. Viruses can cause disease only if they can replicate within body cells. Although IFNs do not prevent attachment and penetration of viruses to host cells, they do stop replication. Some interferons also have important immunoregulatory and anti-tumor effects. There are three families of IFNs; α-, β- and δ-IFN.
The complement system consists of 30 proteins. These proteins are produced by the liver and circulate in the plasma. When activated, the complement proteins collectively can cause cytolysis (bursting) of cells, promote phagocytosis and contribute to inflammation. Most complement proteins are designated by an uppercase letter C and a number; and a few complement proteins referred to as factors B, D and P (properdin). C1-C9 complement proteins act in a cascade. Complement activation may begin by three different pathways: classical pathway requires antibodies bound to antigen, alternate pathway involves factors B, D and P interacting with microbial molecules, and the lectin pathway requires lectin binding on the surface of the microbe. In all three pathways, C3 is activated and splits into fragments C3b and C3a. Although the process of phagocytosis is discussed in another module, C3b enhances phagocytosis by binding to the microbial membrane (opsonization): phagocytic cells have receptors for C3b enhancing the interaction between microbe and phagocyte. C3b fragments together with proteins C5b-C9 initiates a series of reactions that bring about cytolysis through formation of a pore shaped membrane attack complex (MAC) inserted into the microbial membrane. C3a and C5a bind to mast cells and cause histamine release and the resulting increased permeability of blood vessels. C5a also attracts phagocytes to the site of inflammation.
Iron is an important micronutrient most bacteria need to survive. By reducing the amount of iron available, iron-binding proteins called transferrins can inhibit the growth of certain bacteria.
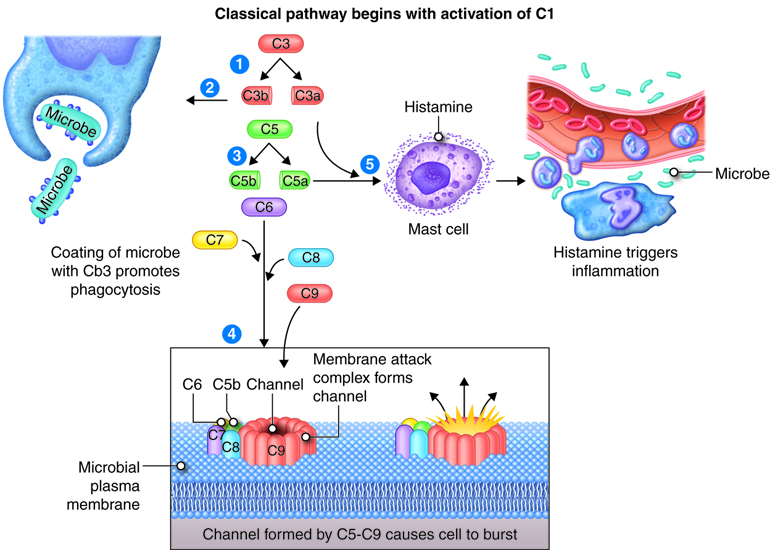
Substances that Contribute to Inflammation
Although the process of inflammation will be detailed in another module, two immediate changes occur in blood vessels in the region of tissue damage and/or infection; vasodilation of arterioles and increased permeability of capillaries. Local immune cells release chemical substances that increase the permeability of the local capillaries. Plasma and leukocytes circulating in the blood can now move through the capillary wall and into the interstitial space. Localized swelling, redness, heat and pain are produced. Among the substances that contribute to these cardinal signs of inflammation are: 1) histamine, 2) kinins, 3) prostaglandins (PGs), 4) leukotrienes (LTs) and 5) complement.
- Histamines are released by mast cells found in connective tissue, basophils found in tissues where allergic reactions are occurring and platelets found in blood in response to tissue injury. Histamine promotes vasodilation and increased permeability of capillaries.
- Kinins form in the blood from inactive precursor proteins called kininogens. In addition to promoting vasodilation and increased permeability, kinins attract phagocytes into inflamed tissues. Bradykinin is an example of a kinin. Kinins affect some nerve endings causing much of the pain associated with inflammation.
- Prostaglandins (PGs) are lipids released by damaged cells that intensify the effects of histamine and kinins. PG may also stimulate movement (chemotaxis) of phagocytes through the capillary wall, regulate smooth muscle contraction and intensify and prolong the pain associated with inflammation.
- Leukotrienes (LTs) are produced by basophils and mast cells and promote increased permeability of capillaries. They also function in chemotaxis and pathogen adherence of phagocytes.
- Complement components (C3b and C5a) stimulate histamine release, function in chemotaxis and phagocytosis.
Molecules Mediating Cell-Cell Recognition
Antigens and Antigen Receptors
Antigens are substances that bind to receptors on immune cells. Entire microbes or parts of microbes may act as antigens. The term antigen is derived from its function as an antibody generator. Chemical components of bacterial structures such as flagella, cell walls and bacterial toxins, viral capsules, pollen, and incompatible blood cells and tissue transplants can all act as antigens. Typically, just small parts of a large antigen molecule act as the triggers for immune responses. These small parts are call epitopes or antigenic determinants. Antigens are most often large, complex molecules such as proteins, however, nucleic acids, lipoprotein, glycoprotein and some polysaccharides may also act as antigens. Simple, repeating subunits, like cellulose and most plastics are not usually antigenic. Antigenicity is the ability to combine specifically with the secreted antibodies and/or surface receptors on T-cells.
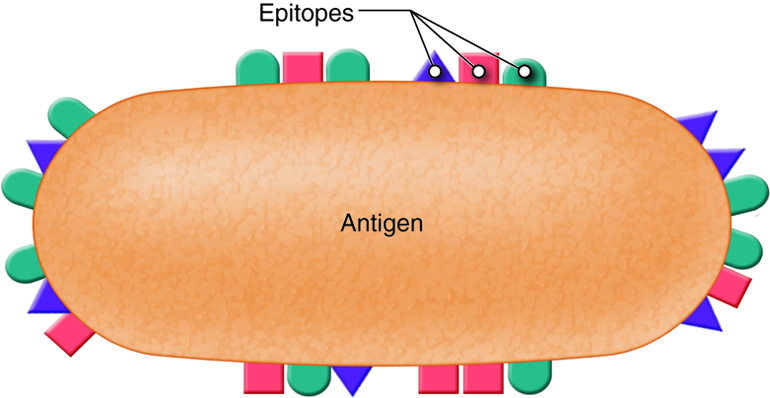
T and B lymphocytes, also called T and B cells, are the mediators of the adaptive immune response. They are lymphocytes with receptors that bind to antigens. Individual T and B cells recognize only one antigenic determinant. This is like a lock and key system where only one key can open the lock. The antigenic determinant is the key. The lock is the receptor on the T or B cell. This T cell receptor (TCR) or B cell receptor (BCR) only fits that one key and opens it (activates it) to initiate an immune. Because T and B cells only recognize one antigen, each T and B cell is said to be antigen-specific.
One feature of the human immune system is its ability to recognize and bind to a least a billion different antigens. Before an antigen enters the body, a small number of T and B cells that can recognize and respond to that intruder are ready and waiting. The diversity of antigen receptors in both T and B cells results from shuffling and rearranging gene segments. This process is called genetic recombination. As the lymphocytes are developing in the bone marrow or thymus, gene segments are put together in different combinations. The situation is similar to having a deck of 52 cards and dealing out three cards. If you did this over and over you could generate many more than 52 sets of three cards. Because of genetic recombination, each T and B cell has now created a unique gene that codes for its unique antigen receptor. Because this random rearrangement of gene segments could produce a TCR or BCR against self antigens, B and T cells must be selected for self-recognition and tolerance. While developing in the bone marrow, B cells that have an antigen receptor that recognizes self-antigens are deleted. T cells that have an antigen receptor that recognizes self-antigens are deleted in the thymus. However, to function properly T cells do not just recognize antigen alone. They must have the ability to recognize antigen in association with their own MHC complex.
Major Histocompatibility Complex (MHC)
Located in the plasma membrane of all nucleated body cells are thousands of transmembrane glycoproteins called major histocompatibility complex (MHC) antigens. Because they were first identified on leukocytes they are also called human leukocyte antigens (HLA). Unless you have an identical twin your MHC antigens are unique. MHC molecules mediate interactions of leukocytes with other leukocytes or body cells. Their normal function is to mark your cells as “self” cells, as T cells will recognize those MHC molecules that do not match your own as foreign. Such recognition can be one of the first steps in any adaptive immune response. Protein molecules are continually made and broken down in a cell. Each MHC molecule combines with a small part of a protein present in the cell (epitope), similar to a hot dog (epitope) in a bun (MHC). The MHC then displays this complex on its membrane surface. The epitope that is presented can either be self or nonself. MHC molecules will become transmembrane molecules only after they associate with an epitope.
The membrane bound MHC fall into two subgroups. Class I MHC (MHC-I) are inserted into the plasma membrane of all nucleated cells of the body. Class II MHC (MHC-II) are only present on antigen-presenting cells (APCs) such as macrophages and B cells.
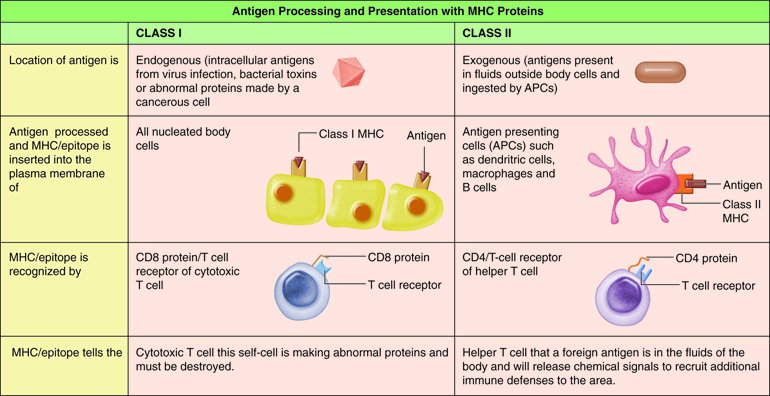
Antibodies
Unlike T cells, B cells do not recognize antigen associated with MHC. Their B cell receptor (BCR) binds to antigen that is circulating freely in the body fluids. Involvement of a B cell in an adaptive immune response is called an antibody-mediated immune response. This is because when activated, B cells produce antibodies.
Antibodies are Y shaped molecules consisting of four polypeptide chains; two identical heavy chains and two identical light chains. The end of each “arm” of the antibody is the variable region and is the part the binds with the antigen with a “lock and key” specificity. The variable region is unique to only those antibodies produced from one activated B cell. Thousands of variable regions exist. The rest of the antibody is the constant region.

Different from the variable region of the antibody, only five different constant regions exist: IgG, IgM, IgE, IgD, and IgA. The constant regions are referred to by the Greek letters gamma, mu, epsilon, delta and alpha. When the region is on an immunoglobulin molecule it is referred to as Ig. Therefore, immunoglobulin is another term for antibody. These constant regions, also called isotypes, are identical between antibodies.
Therefore every different antibody with an IgM constant region will have the same exact IgM constant region, even though the antibodies have different antigen specificity on its variable region. There is no variability in the constant region. Antibodies may exist as single molecules (IgG, IgE and IgD) or may link with another like antibody to form a dimer (IgA) or five like antibodies may join as a pentamer (IgM). Single IgG function well to neutralize, immobilize, clump together, or mark pathogens; dimer IgA are secreted in body fluids; and pentamer IgM activates complement. Each immunoglobulin type is found with different types of infection or location.
The actions of the classes of immunoglobulins differ somewhat, but all of them act to disable antigens in some way. Actions of antibodies include the following:
- Neutralizing antigen of some bacterial toxins and prevents attachment of some viruses to body cells.
- Immobilizing cilia or flagella of motile bacteria thereby limiting their spread into nearby tissues.
- Agglutinating or clumping together pathogens by antigen-antibody cross-linking.
- Activating the classical pathway of the complement system
- Enhancing the activity of phagocytes by “marking” the pathogen as foreign (opsonization)
- Binds to immune cells and stimulates chemical secretion
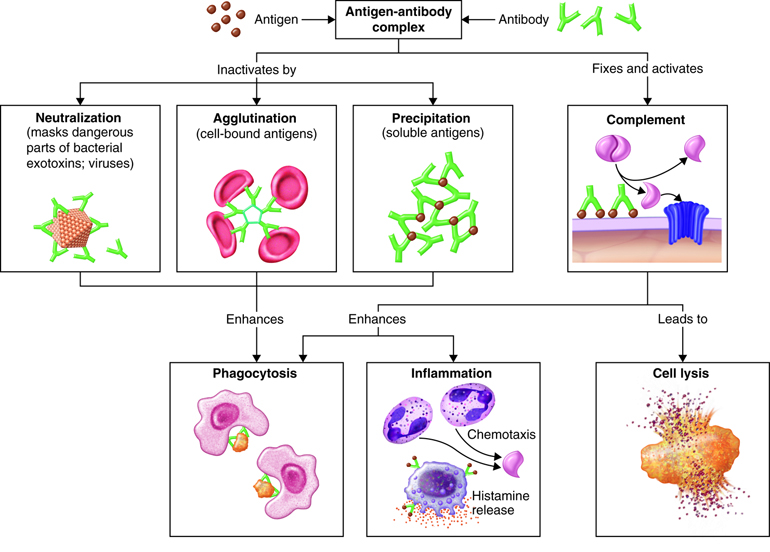
Isotypes | Plasma concentration and immune function |
---|---|
IgM | 5-10% Predominant antibody secreted on first exposure to antigen. Activates complement and agglutinates pathogen |
IgG | 80-85% Predominant antibody secreted on second exposure to antigen. Enhances phagocytosis, neutralizes toxins, activates complement, crosses placenta |
IgA | 10-19% Mainly found in secretions such as tears, saliva, mucus, and breast milk. Neutralizes and immobilizes pathogen |
IgE | Less than 0.1% IgE activate mast cells, basophils and eosinophils. Involved in allergic reactions and provides protection against parasitic infections |
IgD | 0.2% Function not well understood. Present on plasma membrane of B cells and may be involved in their activation |
Proteins Mediating Cell-to-Cell Communication
Low-molecular weight proteins, called cytokines, stimulate or inhibit many cell functions such as cell growth and differentiation. These proteins are secreted by white blood cells and various other cells in the body such as endothelial cells, fibroblasts, liver cells and kidney cells. The two principle regulators of the immune response are helper T cells and macrophages. Cytokines bind to specific receptors on the membrane of cells. Sometimes cytokines bind to receptors in the secreting cell, resulting in self-stimulation. In general, cytokines regulate the intensity and duration of the immune response. Cytokines affect the immune response by stimulating or inhibiting the activation, proliferations and/or differentiation of various cells and by regulating the secretion of antibodies and other cytokines. Some cytokines attract other immune cells to sites of infection, promoting phagocytosis and inflammation.
There are over 200 different cytokines. Cytokines are sometimes named for the cells producing them or for their function. Interleukins are produced by leukocytes and lymphokines by lymphocytes. Chemokines promote movement of cells toward chemicals (chemotaxis). Interferons interfere with viral replication and inflammatory cytokines promote inflammation.
Cellular Level of Organization
Specialized immune cells that reside within the tissues and organs of the lymphatic system recognize foreign pathogens and initiate a local and/or systemic response. These cells are derived from a common precursor cell found in the red bone marrow called the hematopoietic stem cell. These pluripotent hematopoietic stem cells can divide and mature into one of a number of different types of cells. Therefore, they are termed pluripotent, or able to produce a broad variety of cells. This means that many different cells result from the same precursor cell. When cells divide, they mature into different cell types in a process called differentiation. As with all stem cells, the type of cells they differentiate into is controlled by signals in the environment where they reside. Hematopoietic stem cells differentiate into the red blood cells (erythrocytes), platelets and two categories of white blood cells (leukocytes), the myeloid and lymphoid lineages. These leukocytes participate in the immune response and further differentiate into mast-cells, granulocytes (neutrophils, eosinophils and basophils), monocytes (macrophages), dendritic cells and lymphocytes (natural killer cells, B-cells and T-cells).
Cells of the Innate Immune Response
Mast cells
When an infection occurs, local immune cells react and emit signals or chemicals to recruit other immune cells. Among the first to respond are the mast cells that are found surrounding tissues and lymphatic vessels. When bacteria infect tissues, mast cells recognize parts of the bacterial cell wall and release cytokines that recruit neutrophils, eosinophils, basophils and T cells. As part of adaptive immunity, IgE antibodies mediate release of granules, a combination of chemicals that cause vasodilation (histamine, bradykinin), anti-coagulation (heparin), or cell lysis (lysozymes). In this way, mast cell orchestrate allergic responses.
Monocytes and Macrophages
Monocytes circulate in the blood. Chemokines and cytokines signal for the influx of monocytes to a specific area. The cells circulate to the location, migrate into the tissues and differentiate into macrophages. Macrophages reside in almost all tissues. Macrophages engulf pathogens and assist in the immune response by presenting antigenic peptides to helper T cells. Macrophages secrete cytokines that promote inflammation and fever.
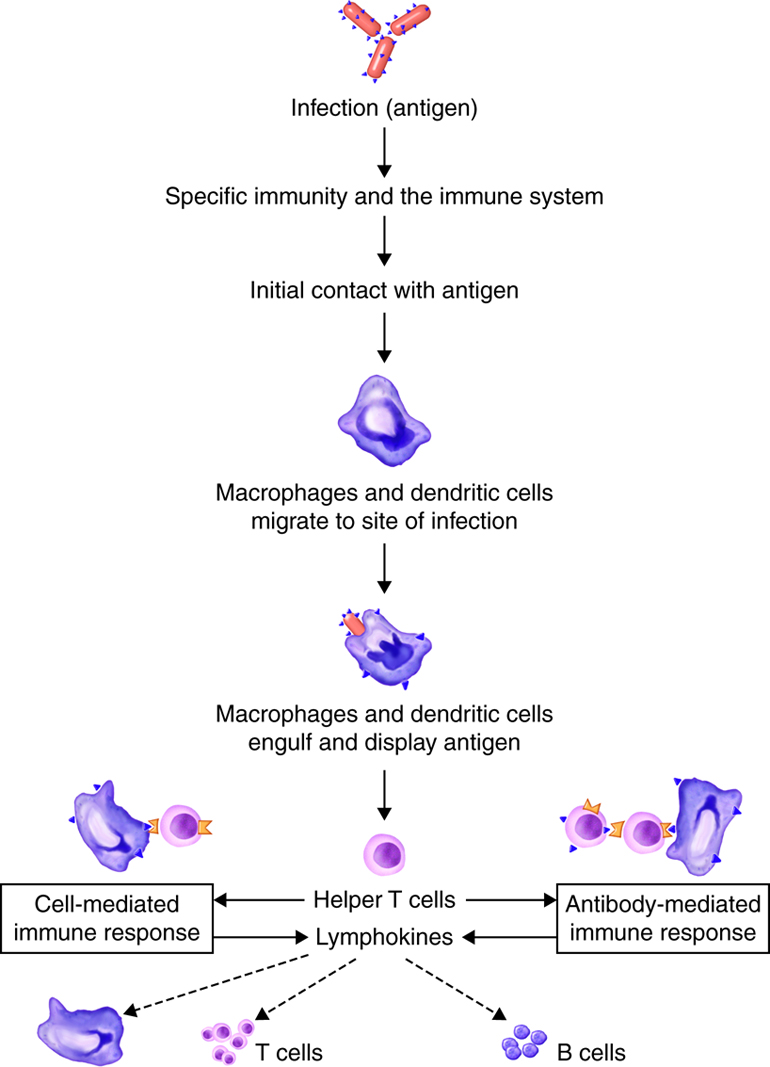
Neutrophils
Neutrophils are the most abundant circulating white blood cell, comprising 50 – 70% of all infiltrating leukocytes. Tissue damage from an infection results in the release of chemokines and other chemical attractant agents called cytokines. These chemokines and cytokines signal for the influx of neutrophils to a specific area. Neutrophils engulf bacteria, digest it and eliminate the infection in a process called phagocytosis.
Eosinophils
Eosinophils target parasitic infections and are abundant in allergic reactions, although eosinophils only constitute a small percentage (1-6%) of leukocytes. The granules of eosinophils include cytotoxic and neurotoxin proteins that can be released to kill multicellular worm parasites. Eosinophils release their toxic and inflammatory mediators through an adaptive immune response. Antibodies (IgE) mediate release of their granules.
Basophils
Basophils are the least abundant circulating white blood cell, constituting only 1–2% of leukocytes. Basophils are recruited by cytokines from the blood to sites of infection. Basophils release histamine, a vasodilator, and heparin, an anti-coagulant, that aid in the infiltration of leukocytes from the bloodstream to site of infection. Basophils are activated through innate receptors and IgE antibodies.
Dendritic cells
Dendritic cells are large, motile cells with long, cytoplasmic extensions. There are several kinds of dendritic cells. These cells migrate through the blood stream from bone marrow and enter tissues. They engulf particulate matter and continually ingest large amounts of extracellular fluid and its contents. Like macrophages and neutrophils, they degrade the pathogens, but their main role is not the clearance of microorganisms. Instead, encounter with pathogen stimulates dendritic cells to activate a particular class of T lymphocytes.
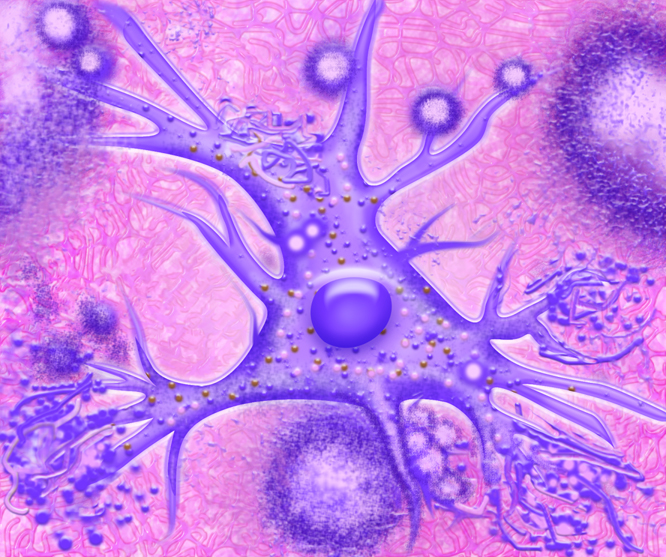
Natural killer cells
Natural killer cells or NK cells are leukocytes that are critically important in the lysis of viral-infected cells. It is thought that NK cells are also able to lyse and eliminate tumor cells. Therefore, many researchers are trying to learn how NK cells function to eliminate tumors in order to design better anti-cancer agents. Binding of NK cells to a target cell, causes the release of granules containing toxic substances. Some granules contain a protein called perforin. These perforin proteins insert into the plasma membrane of the target cell and create holes in the membrane. Extracellular fluid flows through these channels and the cell bursts. Other granules of NK cells release granzymes which induces the tumor or infected cell to undergo self-destruction (apotosis). Unlike traumatic cell death (necrosis), apoptosis is a controlled cell death that produces cell fragments that can be quickly removed before there is damage to the surrounding cells.
Cell | Function |
---|---|
Mast cell | release chemicals to initiate an immune response and orchestrate allergic response |
Macrophages | phagocytosis of pathogen and release cytokines to orchestrate immune response |
Neutrophils | phagocytosis of pathogen |
Eosinophils | destruction of worm parasites and abundant in allergic response |
Basophils | release chemicals to initiate an inflammatory response |
Dendritic cells | phagocytosis and presentation of pathogen as bridge between innate and adaptive immune responses |
Natural killer cells | release chemicals to kill virally infected cells and cancer cells |
Cells of the Adaptive Immune Response
B Lymphocytes
B lymphocytes (B cells) leave the red bone marrow mature and express a unique membrane receptor (BCR) that recognizes a specific antigen. After interacting with antigen, the B cell proliferates and these cells differentiate into specific antibody-secreting plasma cells or memory cells which remain waiting for the next exposure to the exact same antigen.
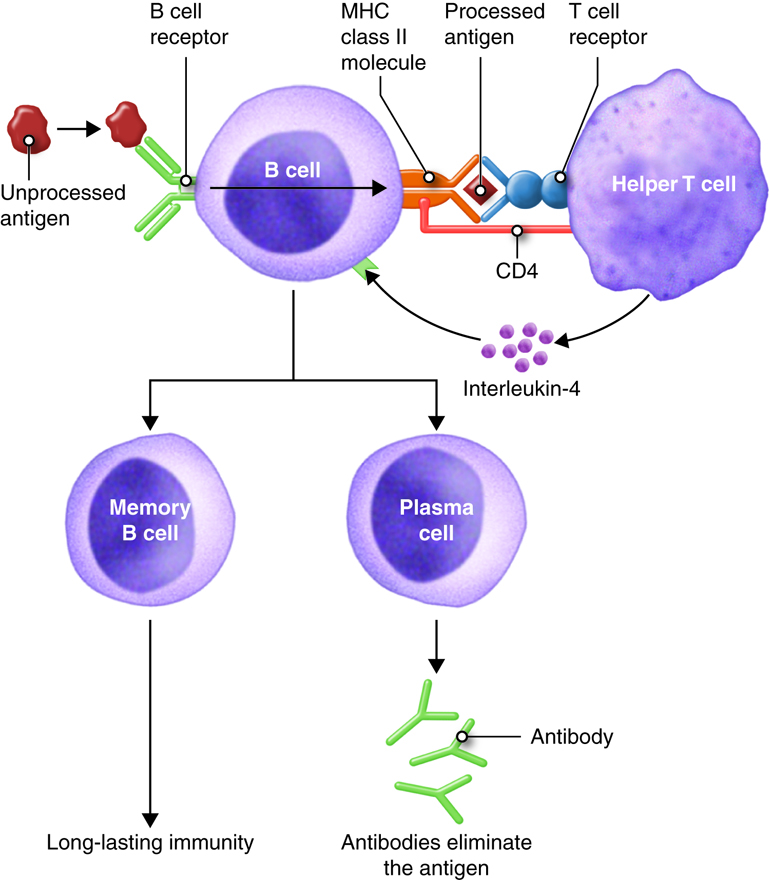
T Lymphocytes
T lymphocytes (T cells) leave the red bone marrow as pre-T cells, mature in the thymus gland and express several distinctive proteins one of which is a membrane receptor. This unique T-cell receptor (TCR) only recognizes a specific antigen that is complexed with a MHC molecule. Several subpopulations of T lymphocytes are recognized such as helper T and cytotoxic T cells. Activated helper T cells provide signals (cytokines) that promote immune responses. There are two subsets of helper T cells. The first subset, Th1, are also called inflammatory T cells and recruit macrophages to sites of infection. The second subset, Th2, are usually referred to as helper T cells and assist B cells in antibody mediated immunity. The cytotoxic T cells destroy target cells when activated.
Antigen Presenting Cells (APC)
Dendritic cells, macrophages and B cells constitue the main APCs. These cells present antigen to helper T cells. APCs can process and present antigenic peptides in association with class II MHC molecules and deliver the co-stimulatory signal necessary for T-cell activation.
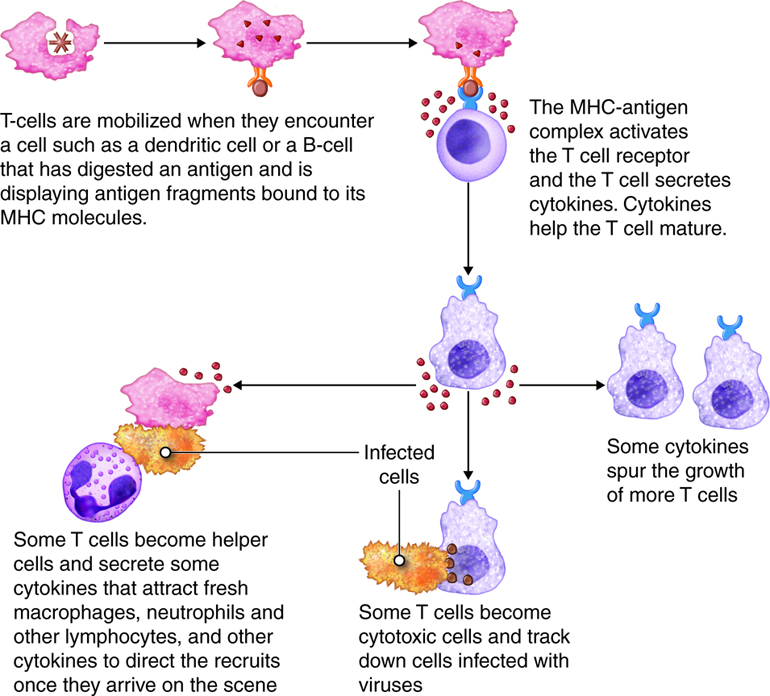
Cell | Function |
---|---|
B lymphocytes (B cell) | Recognize foreign antigen and secrete antibodies |
T lymphocytes (T cell) | Helper T cells provide signals that promote immune responses; cytotoxic T cells kill target cells |
Antigen Presenting Cell (APC) | Present antigen to helper T cells and include dendritic cells, macrophages and B cells |
Tissue Level of Organization
Recall that the lymph and lymph vessels serve as a means of transporting proteins, lipids and pathogens from intersitial fluid to the lymph tissues. Once mature lymphocytes have been generated they circulate in the blood and migrate to lymph tissues where the leukocytes may interact with the trapped pathogen. Lymphatic tissue surrounded by a connective tissue capsule is said to be encapsulated and include lymph organs such as lymph nodes, spleen and thymus. Nonencapsulated lymphatic tissue includes diffuse lymphatic tissue, lymphatic nodules, and the tonsils. Nonencapsulated lymphatic tissue is found in and beneath the mucous membranes lining the digestive, respiratory, urinary and reproductive tracts. In these locations, the lymphatic tissue is strategically located to intercept pathogens that may enter the body.
Diffuse lymphatic tissue has no clear boundary and contains dispersed lymphocytes, macrophages, dendritic cells and other cells. It may be located deep to mucous membranes, around lymphatic nodules and within lymph nodes and spleen.
Lymphoid nodules are denser arrangements of lymphatic tissue organized into spherical structures, ranging in size from a few hundred microns to a few millimeters or more in diameter. Until it is infiltrated by antigen, lymphoid nodules mainly consist of resting B cells and antigen presenting cells (APCs). After antigenic challenge, the nodules consist of antibody-producing B cells (plasma cells) and helper T cells interspersed with macrophages and APCs. Many lymphatic nodules are scattered throughout the connective tissue of mucous membranes lining the respiratory, gastrointestinal, urinary and reproductive tracts. These lymphatic nodules are referred to as mucosa-associated lymphatic tissue (MALT). Although lymph nodules can occur in solitary, many occur in large aggregations. Among these are the Peyer’s patches in the ileum of the small intestine and aggregations in the appendix. Lymphatic nodules may also be located in the lymph nodes and the spleen. In lymph nodes. these nodules receive the name lymphoid follicles, and they contain proliferating B cells called germinal centers.
Tonsils are large groups of lymphatic nodules located deep to the mucous membranes within the pharynx. They are strategically positioned forming a ring in the pharyngeal and oral cavity where they can stimulate immune responses against inhaled or swallowed pathogens. The adenoid is embedded in the nasopharynx, the two palatine tonsils are on either side of the oral cavity, and the paired lingual tonsils are located at the base of the tongue. When infected, tonsils can become inflamed. It is possible to survive without the tonsils.
Organ Level of Organization
The lymphatic system consists of many different tissues and organs that are found throughout the body. Some organs provide the environment for the development and maturation of leukocytes. Other tissues and organs trap pathogen and are the sites where leukocytes can interact with the pathogen. The circulatory and lymphatic systems interact together to connect these organs and tissues.
Several morphologically and functionally diverse organs have various functions in the development of immune responses. These can be distinguished by function as primary and secondary lymphoid organs. The red bone marrow and thymus gland are the primary lymphoid organs, where maturation of B and T lymphocytes occurs. It is in the secondary lymphoid organs and tissues where the immune responses take place. Secondary lymphoid organs include the lymph nodes and spleen.
Bone Marrow
The red bone marrow is the site where hematopoietic stem cells are found. Hematopoietic stem cells differentiate into the red blood cells (erythrocytes), platelets (thrombocytes) and white blood cells (leukocytes). Leukocytes participate in the immune response and further differentiate into mast cells, granulocytes (neutrophils, eosinophils and basophils), monocytes (macrophages), dendritic cells and lymphocytes (natural killer cells, B-cells and T-cells).
After birth, generation of mature B cells occurs in the bone marrow. During B cell development, random gene rearrangement produces an immature or pre-B cell expressing a B cell receptor (BCR). Maturation of pre-B cells involves a positive and negative selection process. Pre-B cells capable of an immune response survive positive selection and cells incapable of an immune response undergo apoptosis. Negative selection causes lymphocytes acting against self-antigens to undergo apoptosis or stimulates them to change their receptor specificity (receptor editing).
Thymus
The thymus is located in the chest, just in front of the heart and behind the sternum of the rib cage. The thymus is comprised of two lobes that are further divided into lobules. Structural thymic tissue consists of epithelial cells joined by desmosomes. This framework of cells form small, irregularly shaped compartments filled with lymphocytes. In addition, the network of epithelial cells surrounds capillaries to form a blood-thymus barrier which prevents the entry of foreign antigens into the thymus. Each lobule consists of an outer cortex and an inner medulla. Immature, pre-T cells (thymocytes) migrate from the red bone marrow to the cortex of the thymus where they mature. Thymocytes pass through a series of stages maked by changes in expression of the TCR and cell-surface proteins such as CD4 and CD8. Epithelial cells in the thymus assist in the maturation process of T cells in the thymic cortex in a process called positive selection. In this process immature T cells express T-cell receptors (TCRs) that interact with class I MHC complexed with self proteins on epithelial cells in the cortex. Cells that recognize the MHC part of the complex survive due to self-recognition. T cells that do not recognize self MHC undergo apoptosis. At the junction of the cortex and the medulla, the development of self-tolerance occurs. The development of self-tolerance occurs in a process called negative selection in which the T cells interact with dendritic cells presenting class I MHC complexed with self proteins. T cells whose TCRs recognize the MHC and also recognize self proteins undergo apoptosis. This process is referred to as clonal deletion. In this way the surviving T cells recognize self MHC (self-recognition) and lack reactivity to self proteins (self-tolerance). The surviving T cells enter the medulla of the thymus and eventually leave the thymus via the blood and migrate to lymph nodes, the spleen and other lymphatic tissues where they colonize parts of these organs. Functionally the thymus continues to mature until puberty. As a person ages the funtional portion of the thymus gland atrophies considerably. However, before the thymus atrophies, it populates the secondary lymphatic organs and tissues with T cells. Like the spleen and tonsils, it is possible to survive without a thymus since the secondary lymphatic organs have already been populated with mature T cells, but the ability to initiate an immune response is diminished.
Lymph Nodes
As the lymph fluid moves from the capillary beds through the lymphatic vessels, and before it flows back into the general circulation it enters into areas of small swellings in the vessels called lymph nodes. Lymph nodes are oval shaped structures that are enclosed in a fibrous outer capsule. Superficial lymph nodes are in the subcutaneous tissue and include inguinal nodes in the groin, axillary nodes in the armpit and cervical nodes in the neck. Deep lymph nodes are everywhere else in the body where there are lymph vessels. Nodes collect and filter lymph from several afferent vessels, and several efferent vessels carry the lymph out from the node. The lymph fluid enters the lymph node and slowly filters through the cortex and medullary regions. Any microbes that were collected in the lymph are detected by the immune cells in the lymph nodes.
Lymph nodes are primarily a site for immune system activation. Specialized immune cells, including B lymphocytes, T lymphocytes, and macarophages, leave the bone marrow or thymus gland and reside within the lymph node. Just inside the fibrous capsule is the outer cortex region that contains the lymphatic nodules, a place where the B lymphocytes encounter antigen and the product of B cells, called antibodies, are generated. Memory B cells persist after an initial encounter in the lymphatic node. The inner cortex (paracortex) contains T cells and dendritic cells that enter a lymph node from other tissues. The dendritic cells present antigens associated with MHC class II to Helper T cells causing their proliferation. At the interior of the lymph node is the medulla, where antibody producing B cells (called plasma cells) are located. Antibodies leave the lymph nodes via the lymph.
Spleen
In addition to lymph nodes, the lymphatic system includes the spleen. The spleen is located in the left, superior corner of the abdominal cavity, just posterior to the stomach, and protected by the rib cage. Although it is about the size of a clenched fist, it is one of the largest immune organ. Shaped like a big lymph node, the spleen similarly contains macrophages and lymphocytes. Instead of lymph flowing through it, the spleen contains blood. In fact, it serves as a reservoir for blood which can be used for a small increase in circulating red blood cells during exercise or emergency situations. Splenic macrophages can phagocytize cellular debris from defective red blood cells. Foreign substances in the blood passing through the spleen can stimulate an immune response because of the presence of lymphocytes.
The spleen consists of two different kinds of tissue called white pulp and red pulp. Blood flowing into the spleen through the splenic arteries enters the central arteries of the white pulp. Within this pulp, B and T cells carry out immune functions similar to lymph nodes. White pulp also contains macrophages that can remove blood-borne pathogens by phagocytosis. Within the red pulp, macrophages can remove worn-out red blood cells and platelets.
It is possible to survive without a spleen if it is damaged. However, removal of the spleen does diminish the ability to mount an immune response.
System Level of Organization
As you have learned, the lymphatic system and immunity are closely related. Immune responses originate in the lymph organs and tissues. The immune system constantly surveys our body for invading bacteria, viruses, or other foreign pathogens. When it encounters a foreign substance, the immune system is activated to destroy the pathogen. Although fully integrated, the immune response is divided into two categories: the innate immune response and the adaptive immune response. The innate immune response is activated immediately (within hours to days) and is always the same. It responds to non-specific cues from the invading pathogens like highly conserved protein peptides that are expressed by many different types of pathogens, and are not expressed on mammalian cells. For example, each time a bacterial cell enters the body it is recognized and phagocytized with the same speed and efficiency. The innate immune response can be so efficient that signs or symptoms of disease never develop.
The adaptive immune response is slow (days to weeks) on first exposure to pathogens, but improves with subsequent exposure. On first exposure, cells of the adaptive immune response identifies a specific pathogen based on the specific proteins in that pathogen and mounts a targeted response to eliminate it. This type of response takes longer to establish and damaged tissues produce signs and symptoms of disease. However, during this time the most important aspect of our adaptive immune response is that it has memory, also called immunological memory. The adaptive immune response produces memory cells so that it is able to recognize foreign substances it has seen before and eliminate the threat both faster and with greater efficiency. Consequently, no signs or symptoms of disease develop and an immunity to that pathogen now exists. Vaccines have been created to take advantage of the immune memory. Because of this, the immune system can be used to prevent many infections and to ward off many diseases, including cancers.
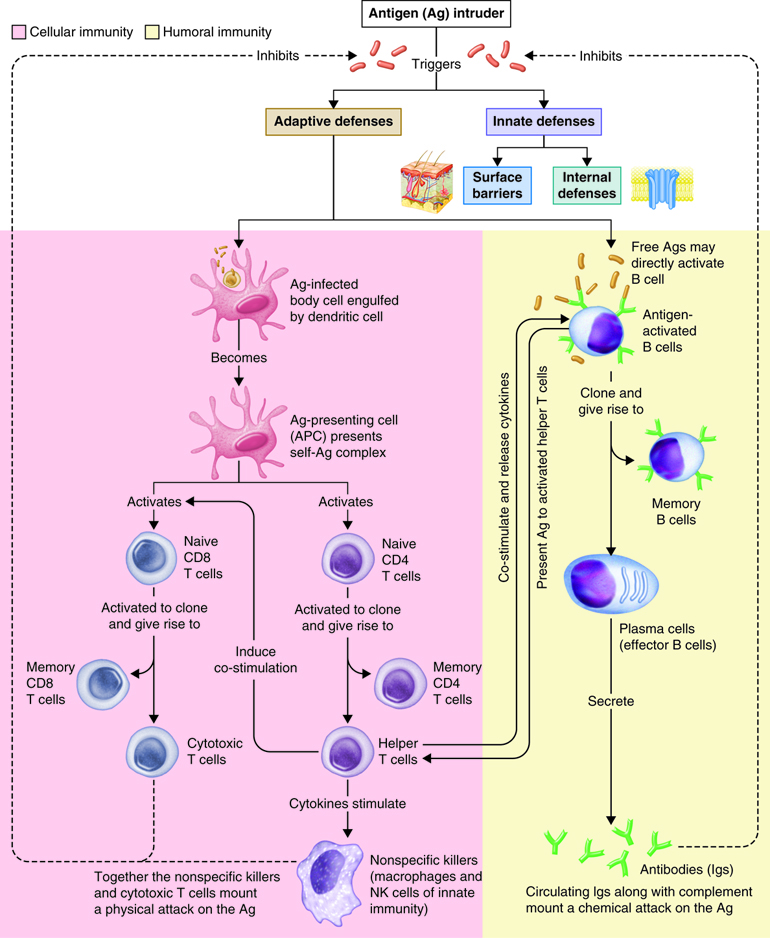
Innate Immunity
Immunity can be seen as a state of protection from infectious disease and has both a less specific innate response and more specific adaptive response. Innate and adaptive immunity are intimately linked. The less specific component provides the first line of defense against infection, most of which fall into the category of innate immunity. Barriers, antimicrobial compounds and phagocytic cells all play important roles in innate immunity and are uniform in all members of a species.
Innate immunity includes mechanisms that are not specific to a particular pathogen but include molecular and cellular components that recognize classes of molecules peculiar to frequently encountered pathogens.
Innate immunity can be seen to comprise five types of defensive mechanisms:
- anatomic barriers
- physiologic barriers
- phagocytosis
- inflammation
- fever
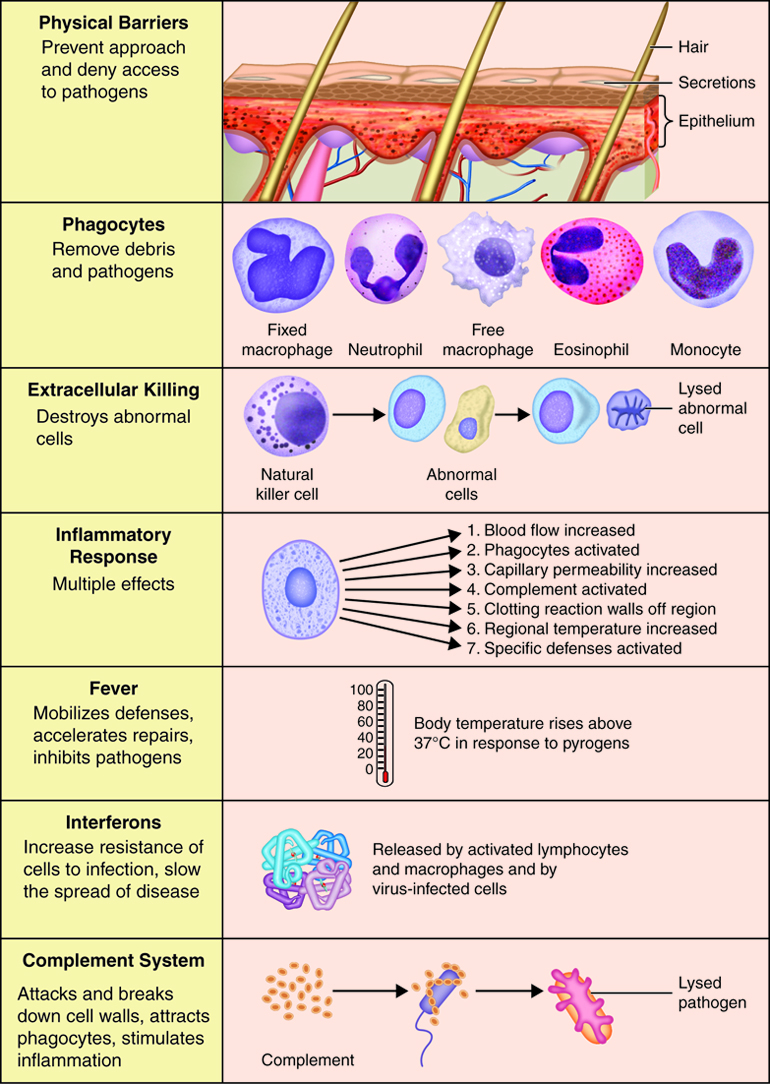
Anatomical and Physiological Barriers
Our immune system is constantly preventing infection by potential invaders. The vast majority of the time we are unaware of any potential threat. The first lines of defense our bodies have against infection are the physical and anatomical barriers. Most infections are avoided because the pathogen cannot penetrate the barriers in place in our body.
The Skin
The largest of the anatomical barriers is our skin. Our skin not only covers and protects the internal organs, it also acts as a shield to prevent infection from invading foreign pathogens. Two layers of cells make up the skin; the innermost layer or dermis, and the outermost layer or epidermis. Within these layers are sweat glands, hair follicles, nerves, and blood vessels that collectively serve to prevent entry into the body, to eliminate excess heat from the body, and to inform the body about the external environment.
Invading pathogens cannot penetrate the epidermal layer of the skin. This makes the skin an incredibly important organ involved with the immune response. Only if the integrity of the skin is broken or damaged can pathogens gain entry. Further, this is only when the wounds are open. Once wound repair has begun and scabbing has occurred, the skin returns to its impenetrable state.
The Brain
Unlike the other organs of the body, the brain does not contain lymphatic vessels. Therefore, the brain lacks a system to filter out invading pathogens. Instead, a physical barrier between the brain and the rest of the body exists. To prevent infection, subset of glial cells in the brain called astrocytes surround the capillaries in the brain to slow or prevent the movement of some proteins and pathogens from the blood into the brain. These cells add another layer that molecules or pathogens have to cross in order to gain access into the brain, called the blood-brain barrier. Because of their size and the thickness of the cell layer, pathogens can rarely cross this barrier. Therefore the brain is protected from infection even though it lacks a lymphatic system. Due to the presence of the blood-brain barrier, the brain is considered an immunologically privileged organ. This means that only certain proteins can gain access to the brain. Sometimes even beneficial medications cannot cross the blood brain barrier.
Physiological Barriers
Potentially infectious pathogens can gain access into the body through any of the openings, including the eyes, ears, nose, mouth, lungs, anus, vagina, or urethra openings. To avoid infection, each of these openings has mechanisms in place to prevent entry. For example, the eyes are protected by the eye lashes, the lid, fluid around the eye, and by tears. Tears not only flush potentially infectious pathogens from the eye, they also contain anti-microbial proteins such as lysozyme.
Ears have small hairs and produce ear wax to trap and remove pathogens. Likewise, nasal passages contain hair to trap pathogens. Nerve endings in the nose can be stimulated by foreign particles, resulting in the sneeze reflex that forcibly blows pathogens from the nasal opening. The mouth secretes lysozymes in response to foreign pathogens. Lysozyme destroys the bacterial cell wall and kills the invading bacteria. If a pathogen successfully evades the barriers and passes through the mouth or nose, it can be trapped in the mucus membrane that lines the lungs. Small microtubules, called cilia, sweep back and forth in a whip-like motion to push the mucus up and out of the lung. This is referred to as the ciliary escalator and helps remove small particles, smoke particulates, and pathogens from the lung. Mucus that exits the lung is usually swallowed and enters the esophagus.
Mucus, tight sphincters, and secretions with low pH reside within the openings of the urogenital tract that prevent infection and kill invading pathogens by physically blocking, trapping, or lysing the cells.
Other physiological events help remove potentially harmful pathogens including vomiting, coughing, diarrhea, and urinating. Because of the high or low pH, and force and speed of expulsion, these mechanisms help flush the body of harmful agents quickly and prevent digestion or absorption through the GI or respiratory system.
Cells in Innate Immunity
Both innate and adaptive immune responses depend on responses of white blood cells. Thesecells all originate in the bone marrow. There are two main lineages of leukocytes, the myeloid and lymphoid. Most of the cells of innate immunityare derived from the myeloid lineage. The common myeloid progenitor is the precursor of the macrophages (the mature form of the monocyte), mast cells, dendritic cells and granulocytes. Granulocytes can further differentiate into neutrophils, eosinophils, and basophils. Collectively, these cells are referred to as the inflammatorycells. Influx of these cells into an infected area produces inflammation thatis characterized by redness, heat, pain and swelling.
When an infection occurs, local immune cells react and emit signals or chemicals torecruit other immune cells. Among the first to respond are the nonmotile mast cells that are found near capillaries in the connective tissue of the skin, lungs, gastrointestinal tract and urogenital tract. Through PPRs, mast cells are stimulated to release cytokines that recruit neutrophils, eosinophils, basophils and T cells.
In addition to mast cells, other specialized immune cells including a group of cells,called the granulocytes, respond to infection. Granulocytes include neutrophils, eosinophils, and basophils.Neutrophils are the most abundant white blood cell, comprising 50 – 70% of all infiltrating leukocytes. Neutrophils are usually the first cells to leave the blood and enter infected tissues in large numbers. They are especially effective in engulfing and digesting bacteria as a way of combating infections. Neutrophils are programmed to die by apoptosis after ingesting bacteria. Pus is an accumulation of deadneutrophils, dead pathogens, tissue debris and fluid. Eosinophils target parasitic infections and are abundant in allergic reactions, although eosinophils only constitute a small percentage (1-6%) of leukocytes. Eosinophils release chemical factors called chemokines. These are chemicals that attract other immune cells andinitiate an adaptive immune response. Basophils are the least abundant, constituting only 1–2% of leukocytes. Basophils release histamine, a vasodilator, and heparin, an anti-coagulant, that aid in the infiltration of leukocytes from the bloodstream to site of infection.
Monocytes circulate in the blood and continually migrate into tissues wherethey mature into macrophages. Macrophages are large motile cells that leave the blood and accumulate in infected tissues after neutrophils. They outlive neutrophils and can ingest more and larger substances than neutrophils can. They are responsible for most of the activity in the late stages of an infection, including the cleanup of dead neutrophils and general debris. Macrophages are also permanent residents beneath the free surfaces of the body and around blood and lymphatic vessels. In these locations macrophages provide protection by trapping and destroying pathogens entering the tissues. Macrophages are waiting within the lymph nodes, spleen, bone marrow and liver as well. An additional role of macrophages is to coordinate immune responses: they induce inflammation, which we will see is a prerequisite to a successful immune response, and they secrete cytokines that activate and recruit other immune system cells into an immune response. Although macrophages can activate naïve T cells through antigen presentation, they often present antigens to T cells to be activated themselves. Some microorganisms, such as mycobacteria, are intracellular pathogens that grow primarily in the phagosomes of macrophages. Peptides derived from such microorganisms can be presented on the macrophage surface by MHC class II molecules. These complexes are recognized by the T-cell receptor of the TH1 subset. The T cells synthesizemolecules that stimulate the macrophage and enable it to eliminate the pathogen. This antimicrobial boost to the macrophage is known as macrophage activation.This coordination between T cells and macrophages results in the formation of the immune reaction call granuloma, in which microbes are held at bay within a centralarea of macrophages surrounded by activated T lymphocytes.
Dendritic cells have almost all of the innate immunity receptors and are extremelysensitive to the presence of pathogens. Stimulation of innate immunity receptors promotes the ingestion and digestion of the pathogen. As part of innate immunity, dendritic cells produce cytokines that attract other immune cells to sites of infection and interferons that inhibit viral infection. Dendritic cells in the skin are called Langerhans cells.
The lymphoid progenitor in the bone marrow gives rise to the antigen-specific lymphocytesof the adaptive immune system and also a type of lymphocyte that responds to the presence of infection but is not specific for antigen, and so it is considered to be part of the innate immune system. These large cells have distinctive granular cytoplasm and are called a natural killer cells (NK cells). These cellsrecognize and kill some abnormal cells, such as tumor cells and cells infected with viruses, and are thought to be important in controlling viral infections until the adaptive immune response has a chance to kick in.
Cellular Physiology of Innate Immunity
Phagocytosis
Phagocytes are specialized cells that engulf and ingest microbes or other particles such as cellular debris. This process is termed phagocytosis. The two main types of phagocytes are neutrophils and macrophages. When an infection occurs, these cells migrate to the infected area. During this migration macrophages enlarge and develop into wandering macrophages. Fixed macrophages stand guard in specific tissues; such as histocytes in connective tissue, Kuppfer cells in the liver and tissue macrophages in the spleen and lymph nodes. In addition to being an innate defense mechanism, phagocytosis by macrophages and dendritic cells plays a vital role in antigen presentation in adaptive immunity.
As part of innate immunity, phagocytosis occurs in four phases:
- Chemotaxis. Chemicals from invading microbes, damaged tissue cells, leukotrienes from basophils and mast cells or activated complement proteins stimulate movement of phagocytes to a site of damage.
- Adherence. Phagocyte attaches to the microbe or other foreign material. Leukotrienes, complement proteins and antibodies bound to the pathogen will enhance adherence (opsonization).
- Ingestion. Plasma membrane extensions (pseudopods) of the phagocyte would engulf the microbe, surround it and internalize the microbe in a sac called a phagosome.
- Digestion. In the cytoplasm the phagosome and vesicles filled with digestive enzymes and lethal oxidants called lysosomes fuse into a larger vesicle called a phagolysosome. This chemical onslaught quickly kills many microbes.
Inflammation
When pathogens breach the epithelial barrier and enter a tissue, they encounter the resident mast cell, macrophages and dendritic cells. The macrophages and dendritic cells phagocytize the pathogen. The macrophage and mast cells release cytokines that attract neutrophils to the site of infection. This is the beginning of a complex innate immune response called inflammation. Inflammation results in the activation and accumulation of immune cells, the release of inflammatory chemicals and the activation of complement.
The four characteristic or cardinal signs and symptoms of inflammation are redness, heat, swelling and pain. Loss of function may also occur at the site depending on the extent of the injury. Inflammation is an attempt to restore tissue homeostasis by removing microbes, toxins or debris at the site of injury and to prepare the site for repair. Inflammation is a normal response to tissue damage and a vital part of tissue repair.
Whether the injury was due to abrasion, burns or viral infection, the inflammatory response has three basic stages:
- Vasodilation and increased permeability of blood vessels. Vasodilation is an increase in blood vessel diameter that allows more blood to flow to the site of injury. Increased permeability permits proteins such as antibodies, clotting factors and complement to enter the injured area because they can leak out of the capillaries.
- Emigration of phagocytes. Emigration is the movement of phagocytes from the blood into interstitial fluid. Phagocytes remove pathogens and dead tissue.
- Tissue repair. In response to injury, mast cells in connective tissue and basophils in blood release histamine. Neutrophils and macrophages attracted to the site of injury also stimulate the release of histamine, which causes vasodilation and increased permeability of blood vessels. Leukotrienes (LTs) produced by basophils and mast cells increase permeability and are chemotactic agents that stimulate emigration. Other substances that contribute to vasodilation and increased permeability are blood kinins and complement components and prostaglandins released by damaged cells. Prostaglandins also intensify and prolong the pain associated with inflammation.
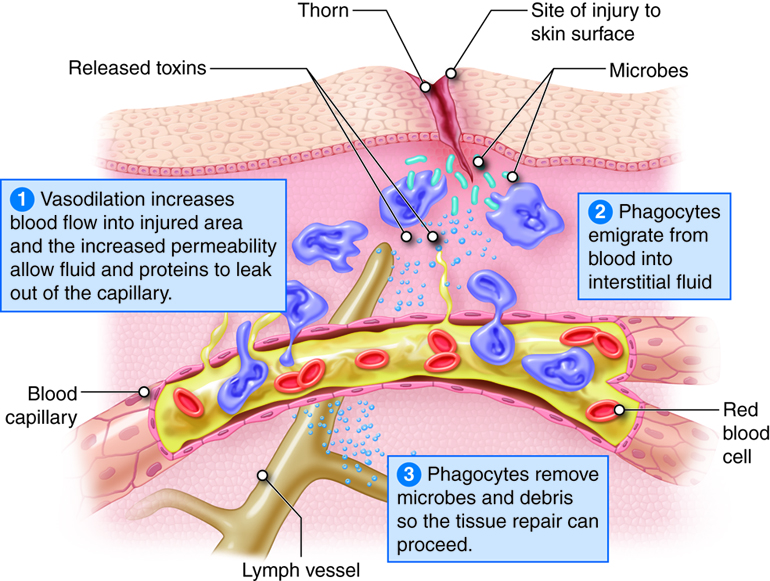
Cell | Function |
---|---|
Mast cell | release chemicals to initiate a allergic inflammation |
Neutrophils | phagocytosis of infected cells and bacteria |
Eosinophils | destruction of parasites |
Basophils | release chemicals to initiate a allergic inflammation |
Natural Killer cells | release chemicals to kill tumor or virally infected cells |
Macrophages | phagocytosis and orchestration of immune responses |
Dendritic cells | phagocytosis and antigen presentation |
Fever
Fevers usually occur in response to infection or inflammation. Fever is an elevated core body temperature (37.2-37.5 C or 99.0-99.5 F in adults) that occurs because a thermostat in the hypothalamus is reset to a higher setpoint. Many bacterial toxins can elevate body temperature by triggering release of fever-causing cytokines (pyrogens) such as interleukin-1 from macrophages. Positive benefits of this elevated body temperature include, inhibiting the growth of some microbes and speeding up chemical reactions involved in the immune response.
The Complement System
The complement system is activated in response to local inflammation. This system can be activated through three different pathways: the classical, alternative, and the MB/Lectin pathways. Activation of any of these pathways results in the creation of a membrane attack complex or MAC. The MAC is made up of 9 proteins that assemble in the presence of an infected cell. They form a complex that can bind to the surface of an invading pathogen or infected cell. MACs are a part of the innate immune response that non-specifically recognize pathogen proteins and bind to the cell surface. When bound, the MAC pokes holes in the membrane of the pathogen and lyses it. Therefore, assembly and activation of the MAC destroys an invading pathogen.
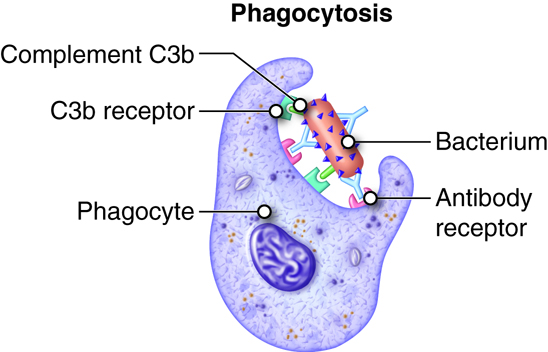
Review of Innate Immunity
The innate immune response system produces a non-specific response to invading pathogens. Immune cells, such as mast cells, macrophages and dendritic cells, recognize protein patterns on the surface of pathogens and mount a localized immune response. Local immune cells release chemical mediators, including chemokines, interferons, and other chemicals that increase the permeability of the local capillaries. These chemicals attract leukocytes, such as neutrophils circulating in the blood, and facilitates their transport across the cell membrane into the tissue. As leukocytes enter the infected area, localized swelling, pain, redness, and heat are produced. These are the hallmark signs of inflammation and may also lead to immobilization of loss of function. Macrophages are also recruited. These are phagocytes that engulf pathogenic organisms, in essence eating the invading pathogens. In addition, non-specific signaling cascades like the complement system are activated. This produces chemical mediators to facilitate influx of leukocytes and assemble the membrane attack complex (MAC) that punches holes in the membrane of the pathogen to lyse the cells.
Adaptive Immunity
Recall that immune cells that are part of the innate immune response have receptors that recognize molecules on the surface of invading pathogens that are common to many different pathogens. Since they are common, they also have not significantly changed over thousands to millions of years. Some pathogens, however, have developed mechanisms to protect the molecules on their outer membranes and shield them from the immune system. For example, some bacteria and viruses have added an extra layer to their outer protein coat. This coating helps the pathogen evade detection by the phagocytic cells of the innate immune system.
Adaptive responses are initiated when antigen and antigen-presenting cells, particularly dendritic cells presenting antigens picked up at sites of infection, enter into secondary lymphoid tissue. Dendritic cells have pattern recognition receptors that recognize molecular patterns common to microorganisms. Binding to receptors stimulates dendritic cells to engulf and degrade the microbe intracellularly. Dendritic cells can also take up extracellular material, including viruses and bacteria in a manner that is receptor-independent. The dendritic cells then migrate to secondary lymph tissue and present this antigen to T lymphocytes. This process will be discussed in more detail in a later module. Free antigen can also stimulate antigen receptors of B cells, but most B cells require help from activated helper T cells. The activation of naïve T lymphocytes is therefore an essential first step in adaptive immune responses. For the adaptive immune response to occur, naïve lymphocytes first have to recognize antigens. After recognition, the naïve lymphocytes divide and differentiate, producing a large number of lymphocytes capable of responding to the antigen.
The innate and adaptive immune responses have two important differences. First is time. Recall that most components of innate immunity are present before the onset of infection. In contrast, adaptive immunity does not come into play until after the onset of infection. Adaptive immunity responds with a high degree of specificity. Because the adaptive immune response is initiated in response to the recognition of a specific part of a pathogen, it takes 7-10 days to mount an effective response. Contrast this to an innate immune response that is established immediately, and enhanced within minutes to hours. The second difference is the creation of immunological memory. Adaptive immune responses remember that a specific pathogen has been detected before. When the pathogen is encountered again, the immune response that is elicited is both faster and more robust.
T Cells, B Cells, and Clonal Expansion
T and B lymphocytes are the mediators of the adaptive immune response. T and B cells are able to identify a specific pathogen and initiate an immune response. Any molecule that can bind specifically to an antibody or generate peptide fragments that are recognized by a T-cell receptor is called an antigen. Lymphocytes do not recognize an entire antigen. Antigenic determinants, or epitopes, are specific regions of a given antigen to which an antibody or antigen receptor will bind. Because antigens are larger molecules a variety of epitopes could be found on a single antigen. Each T and B cell recognizes only one epitope. All the lymphocytes of a given clone have identical antigen receptors, which combine with a specific epitope. This is like a lock and key system. The pathogen has a protein that is broken down into a protein fragment (peptide). This peptide is the epitope that acts like a key that only fits one lock. That lock is the receptor on the T or B cell. When the epitope (key) is inserted into the matching T cell receptor or B cell receptor (lock) it initiates a series of events (turning the key) which activates the immune response that will affect the microbe expressing that one epitope. Because T and B cells only recognize one antigen, each T and B cell is said to be antigen-specific. In order to recognize all the different antigens, thousands and thousands of different T and B cells are created and circulate in our bodies. If a T or B cell encounters its target pathogen, then that T or B cell becomes stimulated and begins to rapidly divide. Each mitotic division produces an exact copy of the parent cell (clone). Thousands of clones are created in a process called clonal expansion. This produces thousands of daughter cells that all have receptors specific for the same epitope. Adaptive immunity occurs later than innate immunity because the few B cells and T cells specific for the pathogen must first undergo clonal expansion before they differentiate into effector cells and migrate to the site of infection.
A naïve lymphocyte is a mature helper T cell, cytotoxic T cell or B cell that has not yet bound to the epitope to which its receptor is specific. Naïve lymphocytes continually pass through peripheral lymphatic tissues where they may encounter an antigen. For example a naïve lymphocytes can pass from one lymph node to blood and be carried to another peripheral lymhatic tissue. Naïve T and B cells continuously circulate in the blood, the tissues, and the lymph vessels and nodes. When they encounter and recognize a pathogen within the lymph system, they stop circulating and begin to clonally expand.
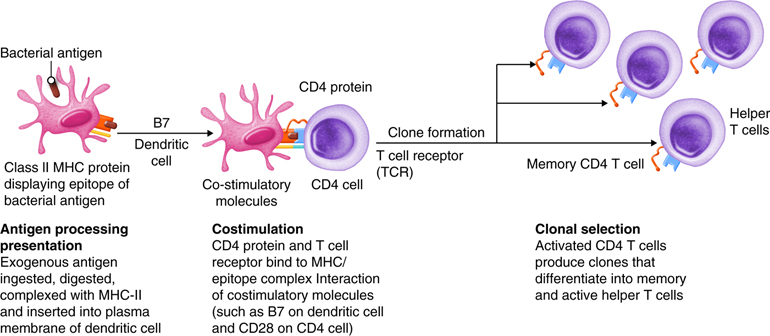
Cell-Mediated Immune Response
Antigens are brought from infected tissues to peripheral lymphatic tissues by dendritic cells, which capture antigens in two ways. Stimulation of innate immunity receptors on the dendritic cell by the pathogen promotes phagocytosis by the dendritic cell and the display of antigens with MHC class II molecules. It is also possible that the dendritic cell is infected by an intracellular pathogen, such as a virus, which results in antigen display by MHC class I molecules. In response to the antigen display with MHC, dendritic cells become mobile and move along with lymph to the nearest lymphatic tissue, where they present the antigen to naïve lymphocytes.
Antigens in lymph and blood can also enter peripheral lymphatic tissues and stimulate the innate immunity receptors of macrophages, which phagocytize the antigens and display epitopes with MHC class II molecules.
Dendritic cells and macrophages expose naïve T cells to their epitopes, resulting in their activation. Activated helper T cells assist in the activation of B cells and cytotoxic T cells.
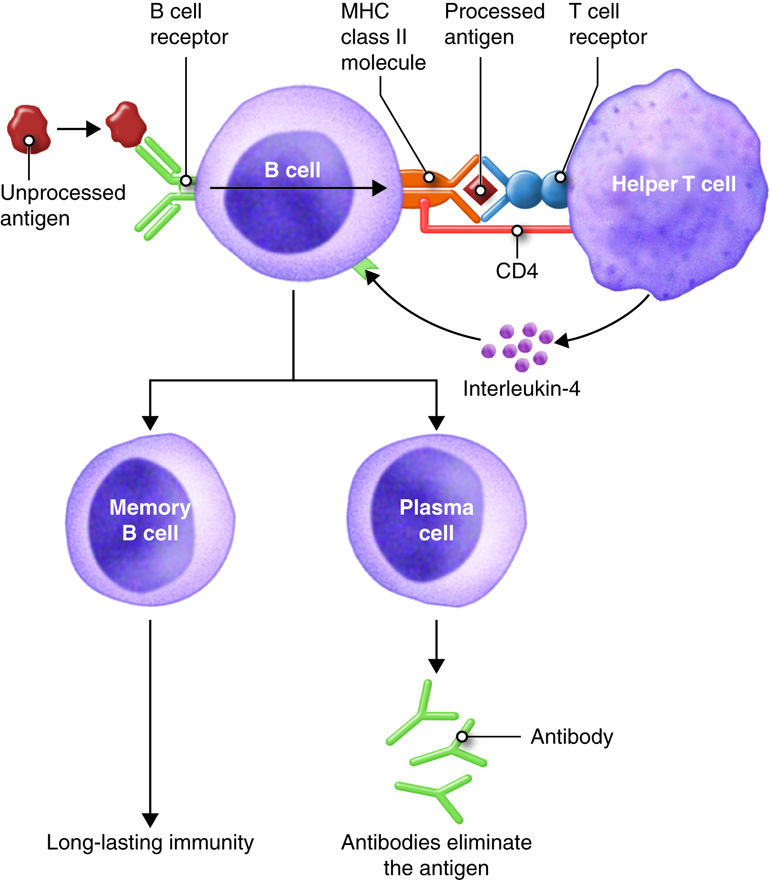
Antigen Processing and Presentation
T cells only recognize fragments of antigenic proteins that are processed and presented by specific immune cells. In antigenic processing, antigenic proteins are broken down into peptide fragments that then associate with MHC molecules . Next the MHC:epitope complex is inserted into the plasma membrane of a body cell. The insertion of the complex into the plasma membrane is called antigen presentation. Antigen processing and presentation occurs in two ways depending on whether the antigen is located intracellular or extracellular.
Processing of Exogenous Antigens
Foreign antigens present in fluids outside body cells are termed exogenous antigens. Examples include intruders such as bacteria and bacterial toxins, viruses that have not yet infected a cell, parasitic worms, and inhaled pollens. Antigen-presenting cells (APCs) process and present exogenous antigens. They are located in places where antigens are likely to penetrate the innate barriers and enter the body. Such places include the the skin (for example, Langerhans cells are dendritic cells) and mucous membranes that line the respiratory, gastrointestinal, urinary and reproductive tracts. After processing antigen, APCs migrate from tissues via lymphatic vessels to lymph nodes where they will present their MHC:epitope complex to T lymphocytes.
The steps in the processing and presenting an exogenous antigen by an APC occur as follows:
- Ingestion of the antigen by phagocytosis or endocytosis.
- Digestion of antigen into peptide fragments within the phagosome or endosome by protein-digesting enzymes.
- Synthesis of class II MHC molecules (MHC-II) in the endoplasmic reticulum.
- Packaging of MHC-II molecules into vesicles by the Golgi complex.
- Fusion of the phagosome or endosome containing the antigen fragments with the vesicle containing the MHC-II.
- Binding of the peptide fragment to MHC-ll molecules.
- Insertion of the MHC-II/epitope complex into the plasma membrane of the APC by exocytosis.
It is important to note that exogenous antigens are always enclosed in vesicles when they are brought into the cell. In this way normal cellular peptides are not complexed with class II MHC, only foreign peptides. After processing an antigen, the APC migrates to lymphtic tissue to present the antigen to helper T cells. This informs T cells that specific intruders are present in the body and that immune responses should begin.
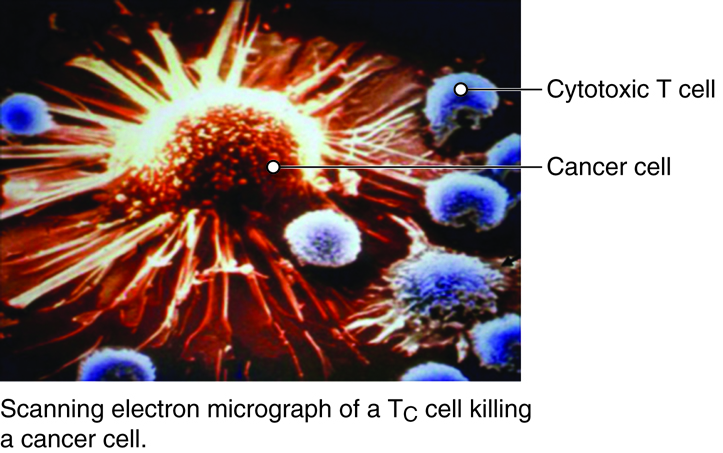
Processing of Endogenous Antigens
Antigens that are present inside the body cells are called endogenous antigens. All nucleated cells of the body have the ability to present antigens complexed with a class I MHC molecule. When a peptide fragment comes from a self-protein, T cells ignore the MHC-I/epitope complex. However, if the peptide fragment comes from a foreign protein, T cells recognize the MHC-I/epitope complex and an immune response occurs. Such foreign antigens may be viral proteins produced after a virus infects the cell and takes over the cell’s metabolic machinery, toxins produced from intracellular bacteria, or abnormal proteins synthesized by a cancerous cell.
Most cells of the body can process and present endogenous antigens. The display of an endogenous self-antigen bound to an MHC-I molecule signals that a cell is normal, however, an endogenous foreign antigen bound to a MHC-I signals that a cell has been infected or is abnormal and needs attention. Cytotoxic T cells have receptors for foreign MHC-I/epitope complex.
Activation of T Cells and Immune Responses
T lymphocytes come in several varieties. They are helper T (Th) cells and cytotoxic T cells (Tc). Each type plays an important role in the adaptive immune response. Helper T cells bind to cells and release cytokines needed for coordinating virtually all immune responses. Cytotoxic T cells bind to cells and lyse them to destroy them. There are several subsets of cytotoxic and helper T cells that employ different techniques to recognize and eliminate pathogens. Ultimately T cells all function to specifically recognize and destroy pathogens associated with a self-cell and by recruitment of immune cells, hence the term cell-mediated responses.
Most mature T cells are naïve because they have not yet encountered a pathogen. However, when an antigen enters the body only a select few T cells have T-cell receptors (TCRs) that can recognize and bind to that specific antigen. Recall that TCRs recognize and bind only to foreign antigen fragments that are presented in MHC/epitope complex. Antigen recognition also involves other surface proteins on T cells.
Inactive helper T cells recognize exogenous antigen fragments associated with class II MHC molecules on the surface of APCs. With the aid of the CD4 proteins, a subset of helper T cells becomes activated. Once activated the CD4+helper T cell undergoes mitotic divisions forming identical copies of itself (clones). This proliferation and differentiation of T helper cells produces both active helper T cells and memory helper T cells. Within hours of activation, active T helper cells start secreting cytokines. Cytokines secreted by these helper T cells act as costimulators for resting helper T cells or cytotoxic T cells, and these cytokines also enhance activation and proliferation of B cells, macrophages and natural killer cells. Memory helper T cells are not active during this current immune response, however, if the same antigen enters the body again, many memory helper T cells can rapidly proliferate and differentiate into active helper T cells and memory helper T cells.
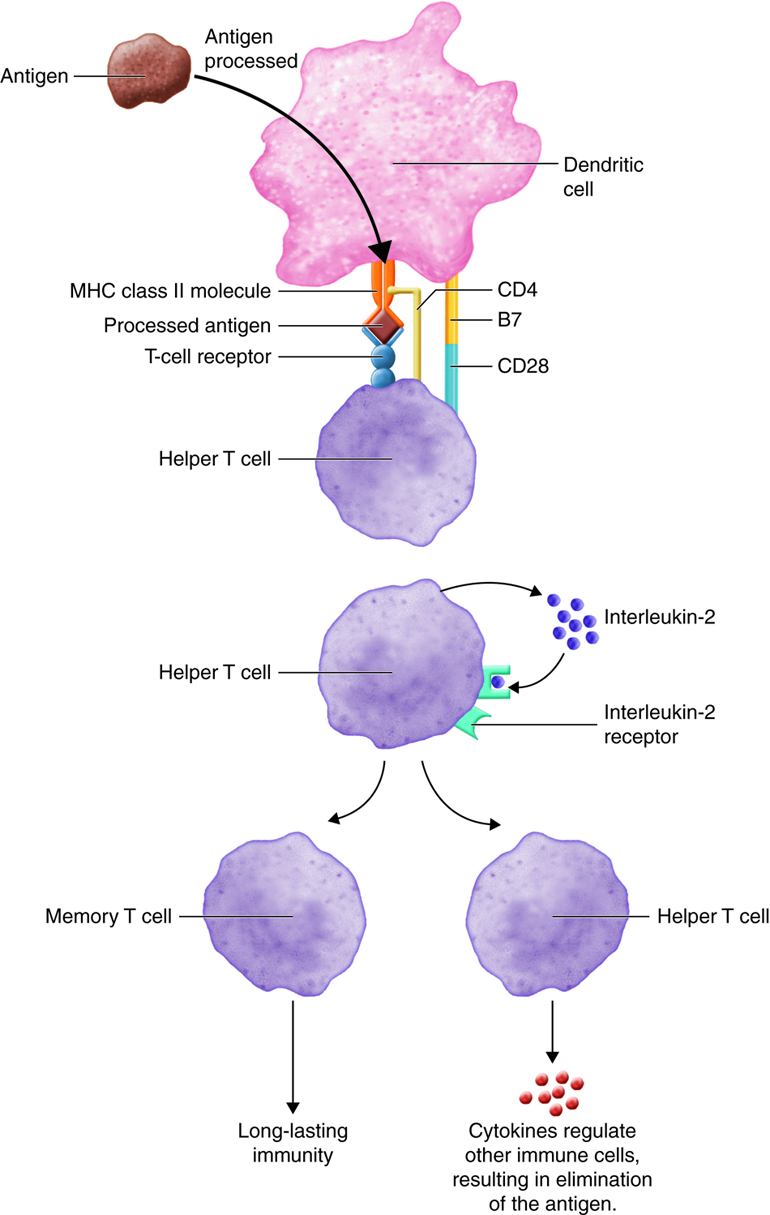
Most T cells that display CD8 glycoproteins develop into cytotoxic T cells. Cytotoxic T cells are most effective against pathogens that live inside the cells of the body. Cytotoxic T cells recognize endogenous antigen fragments associated with class I MHC molecules displayed on the surface of nucleated cells (target cells) infected with a pathogen, some tumor cells displaying tumor antigens, or cells of a tissue transplant.
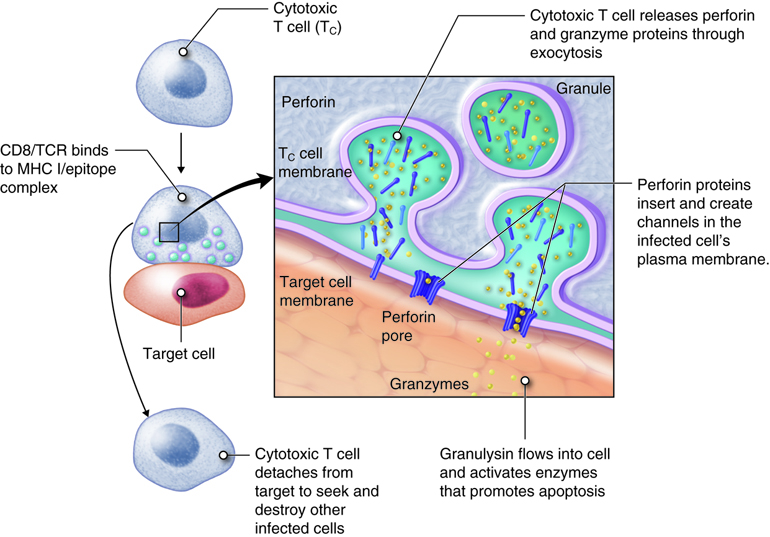
Once activated, a cytotoxic T cell recognizes a target cell infected with intracellular pathogens through its TCR, which binds to MHC-I/epitope complexes on the target cell. The cytotoxic T cell binds to the target cell and releases perforin and granzyme proteins on to the surface of the target cell. The perforin inserts into the plasma membrane of the target cell and creates channels in the membrane. This allows extracellular fluid and granulysin to flow into the cell causing bursting of the cell (cytolysis). Additional granzyme molecules released by the cytotoxic T cell activate enzymes in the target cell that cause fragmentation of its DNA and apoptosis. Cytotoxic T cells also secrete gamma-interferon that attracts phagocytes to the site. After detaching from the target cell, a cytotoxic T cell can continue to seek and destroy other target cells. Memory cytotoxic T cells are inactive during this immune response but can proliferate and differentiate into active cytotoxic T cells if the infection returns.
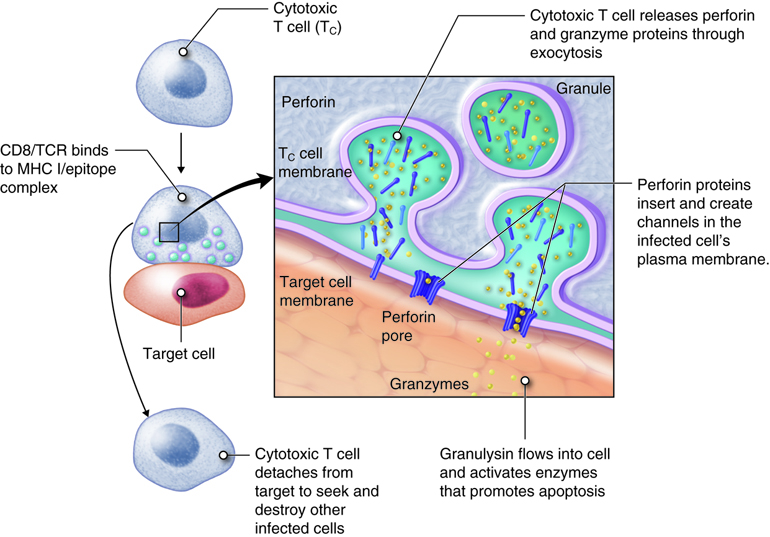
Antibody-Mediated (Humoral) Immune Response
Antibody mediated immunity works mainly against extracellular pathogens, which include any bacteria, fungi or viruses that are in the extracellular fluids of the body. Antibody-mediated immunity involves antibodies that bind to antigens in body fluids. Cytotoxic T cells leave lymphatic tissues to seek out and destroy a foreign antigen, however, B cells remain in lymphatic tissues. In the presence of antigen, B cells in mucous associated lymph tissue (MALT), lymph nodes or spleen become activated. Although B cells can respond to an unprocessed antigen present in lymph or interstitial fluid, their response is more intense if they process the antigen and present it to helper T cells.
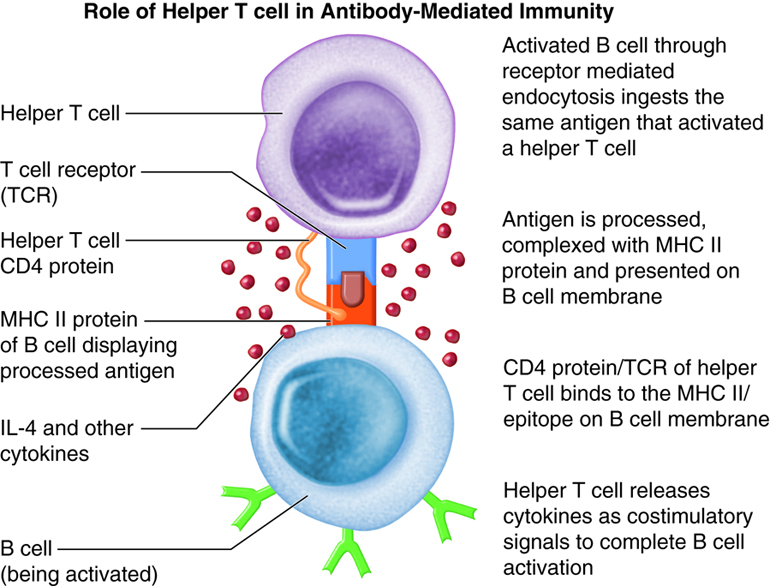
The requirement for T-cell help means that before a B cell can be induced to make antibody against the molecules of an infecting microbe, CD4+ T cells specific for peptides from this microbe must be activated to produce helper T cells. The helper T cell secretes interleukins, especially IL-4, which stimulates the B cell to proliferate identical copies of itself (clones) that differentiate into antibody producing plasma cells and memory B cells. Memory B cells that encounter the same antigen in the future results in a rapid proliferation and differentiation of memory B cells into antibody-producing plasma cells and new memory B cells.
All of the plasma cells of a particular clone are capable of secreting antibodies specific for the antigen that originally stimulated the BCR of the B cell. IgM is the first antibody secreted by activated B cells, but makes up less than 10% of the immunoglobulin found in plasma; IgG is the most abundant. Much of the antibody in plasma has therefore been produced by plasma cells derived from B cells that have undergone isotype switching. Recall that the isotype refers to the constant region of the antibody and determines the action that the antibody takes to defend the body from this pathogen. So the early stages of the immune response are dominated by IgM antibodies, later IgG and IgA predominate, with IgE contributing a small but important part of the immune response.
Immunological Memory
The most significant difference between an innate and adaptive immune response is immunological memory. This process only occurs with adaptive immunity. Innate responses do not produce any memory cells. Therefore, each and every time an innate immune response is initiated, new cells are recruited and the same degree of response occurs. When an adaptive response is initiated and the T and B cells proliferate, specialized memory cells are created along with the effector cells. Now, present in the tissues, are many long-surviving cells able to recognize the antigen. If the antigen enters the body a subsequent time, a rapid and more robust immune response occurs.
The first time the adaptive immune system encounters an antigen, the primary response will take 5 –7 days before clonal expansion is complete and the lymphocytes have differentiated into effector cells. During the initial primary exposure IgM is the most prominent antibody secreted. The second and all subsequent times that the adaptive immune system encounters this same antigen, the immune response will only take 1–3 days to reach its maximal level and IgG is the prominent antibody secreted. Memory cells can live for years and even decades. Therefore, as long as memory T or B cells are circulating in the body, a faster, more robust immune response can be established. This helps to eliminate the infection faster and more efficiently.
It is possible to acquire the immunological memory of adaptive immunity naturally or artificially. Natural immunity results from natural exposure to an antigen. For example, someone sneezes the influenza virus into the air and then it is breathed it into another person’s nasal passageway. Although not always, but symptoms of the exposure usually develop because the person is not immune during the first exposure. In artificial immunity, an antigen is deliberately introduced into an individual to stimulate an immune response. This introduced antigen is a vaccine and the process is vaccination. Both of these immune responses are considered active processes and can persist for weeks to a lifetime because memory cells have been produced. Passive immunity is not long-lasting because the individual does not produce memory cells. Instead, this temporary immunity results from the transfer of antibodies from a donor to a recipient. An example of passive immunity results from a mother to her child as antibodies move across the placenta before birth.
Example: Clinical Connection: Vaccines
Without immunological memory, vaccines would not work. Vaccines can prevent or decrease the severity of infections. The mechanism underlying vaccination is that exposure of the adaptive immune system to a small dose of an antigen will produce an initial immune response. More importantly, this small dose of antigen establishes a population of memory T and B cells that will live long-term in the body. When that antigen is encountered again, the immune system is already primed. Upon a subsequent exposure, a more robust and faster response is established and the pathogen is destroyed faster. Thereby preventing clinical manifestations of the infection or greatly reducing the clinical manifestations.
There are two types of vaccines commonly used: live-attenuated (viable) vaccines and killed vaccines. Live-attenuated vaccines are living organisms that have been modified in such a way that they will grow poorly in the human host and will therefore produce immunity but not disease. People who receive the flu vaccine may have mild flu-like symptoms for a few days but do not get the symptoms of disease that may last for two weeks or more. Killed vaccines have been killed, usually by irradiation, prior to administration. Both types of vaccination contain antigenic proteins that will create memory cells to protect the person from future infection.
Typically, use of live but weakened microorganisms in vaccines produces a more robust immune response than does killed vaccines. Until now, the underlying reason why has remained unknown. Recently, however, it was discovered that immune cells are able to recognize whether a bacterium is live-attenuated or not (Sander et al, 2011). The presence (and detection) of prokaryotic mRNA signals to the immune system that the invading bacteria is live and, therefore, potentially infectious. As a result, a strong immune response is initiated. It is not just presence of prokaryotic mRNA, however, but specifically, the immune cells recognize a lack of the 3’-polyA tail on the prokaryotic mRNA. This means that vaccines that are augmented with prokaryotic mRNA might be able to produce an enhanced immune response in the future. This could have a significant impact on vaccine design and development.
Example: Clinical Connection: Self-Recognition and Self-Tolerance
If cells are created to attack a foreign antigen, how does the immune system avoid attacking itself? The immune system produces a powerful response to foreign pathogens, but does not attack its own cells or molecules. To avoid attacking self, several mechanisms have been established. The phagocytic cells can differentiate between pathogenic organisms (bacteria, viruses, parasites and fungus) and human cells. Pathogens express pathogen-specific signatures (PAMPs) that are not found on mammalian cells. During development, B and T cells that recognize self-proteins are eliminated during a process called clonal deletion. If a T cell does bind to its antigen, but does not receive the proper costimulatory molecule stimulation, the T cell is rendered inactive or tolerant. The T cell is anergic and unable to properly initiate an immune response.
Both the innate and the adaptive immune responses have mechanisms in place to avoid the destruction of their own tissues. Rarely, these mechanisms go awry and an immune response to self-protein occurs. When they do malfunction, auto-immune diseases arise. These are diseases where the immune system considers one’s own molecules as foreign. Most of these diseases have a genetic component to them (they tend to run in families), but also may require an environmental trigger.
Review of Adaptive Immunity
Unlike the innate immune response, the adaptive immune response responds to a specific pathogen. During the adaptive response, B cell receptors recognize pathogens free in body fluids. Additionally, these B cells and dendritic cells engulf and digest the pathogen into peptide fragments. These peptides are bound to a cellular protein called the major histocompatability complex (MHC) and carried together to the surface of the phagocytic cell. This process is called antigen presentation. These peptides presented in the context of (bound to) MHC proteins are recognized by helper T cells. The helper T cell receptors bind to the peptide and MHC protein and become activated releasing cytokines. Cytotoxic T cell receptors recognize foreign peptides that were produced in the cell and associated with MHC such as occurs in infected or tumor cells. Activated T cells, called lymphoblasts, and activated B cells, called plasma cells, begin to divide, creating hundreds of clones of the antigen-specific T or B cell. These clones mount a cellular-mediated immune response or an antibody-mediated immune response to rid the body of the pathogen. Memory T and B cells are also created, which remain in the body and initiate an immune response if the antigen is detected again. Vaccines are effective because of memory cells.
