Chapter 6: Establishing the Postmortem Interval and Forensic Taphonomy
POSTMORTEM INTERVAL
One of the pieces of information it is imperative to determine when human remains are discovered is how long this individual has been dead. This is called the time since death or the postmortem interval. Knowledge of the PMI can aid in identifying the remains and refuting or confirming the alibis of suspects.9
A pathologist will make an estimate of the Postmortem Interval (PMI) by observing evidence of four common post-mortem (after-death) indicators. These are body temperature, the degree of rigor mortis, post-mortem lividity, and progress of decomposition.9
Body Temperature
Algor Mortis is the scientific name given to the loss of body temperature after death which can sometimes be used to estimate the time of death. This is a viable technique in cases where the body is being examined within 24 hours following death. This method of estimating time of death can vary significantly dependent upon many possible variables, such as:
Ambient room temperature being within a normal range of approximately 22° Celsius,
Pre-death body temperature of the victim not being elevated by illness or exertion,
Thickness of clothing that might insulate the body temperature escape, and
Outside temperature and/or conductivity caused the temperature of the body to artificially increase or decrease.9
Considering a normal body temperature of 37° Celsius (98.6˚ F) at the time of death, it can be estimated that the body will cool at a rate of 1° – 1.5° Celsius per hour. This calculation is known as the Glaister Equation. So, taking an internal rectal temperature and subtracting that from 37° Celsius will provide an estimate of the number of hours that have passed since the time of death. For example, a dead body with a measured temperature of 34° Celsius would provide a time range of 3 to 4.5 hours since the time of death.9
Rigor Mortis
Rigor mortis is a term used to describe the stiffening of the body muscles after death.9 A dead body will go from a flaccid or limp muscle condition to one where all the muscles become contracted and stiff causing the entire body to become constricted into a fixed position. ⁹ This occurs because of a lack of ATP being produced.
ATP or adenosine triphosphate is classified as a high energy compound.5 In the body, the energy released from this high energy compound helps fuel the body’s activities, from muscle contraction to the transport of substances in and out of cells.5 Without ATP muscles cannot relax.
After being in a constricted and fixed position, the muscles eventually become flaccid again as muscles begin to decompose. In normal room temperatures, this stiffening of muscles and the relaxing again has a predictable time progression of approximately 36 hours. In this progression, the stiffening of muscles will take approximately 12 hours, the body will remain stiff for 12 hours and will progressively become flaccid again over the next 12 hours.9
Stiffening of muscles begins with the small muscles of the hands and face during the first 2 to 6 hours, and then progresses into the larger muscle groups of the torso, arms, and legs over the next 6 to 12 hours. These are general rules; however, the rate of rigor mortis can be different for infants, persons with extreme muscle development, or where extensive muscle activity precedes death, such as a violent struggle.9
In determining the time of death in average environmental temperature it is recommended that:
- If the body feels warm and is flaccid, it has been dead for less than 3 hours,
- If the body feels warm and is stiff, it has been dead for 3 to 8 hours,
- If the body feels cold and stiff, it has been dead for 8 to 36 hours, and
- If the body feels cold and is flaccid, it has been dead more than 36 hours.9
Post-Mortem Lividity
Post-mortem lividity refers to a discoloration or staining of the skin of a dead body as the blood cells settle to the lowest part of the body due to gravity. This discoloration will occur across the entire lower side of a body; however, in places where parts of the body are in contact with the floor or another solid object, the flesh compresses and staining will not occur in that area. The staining is a reddish-purple coloring, and it starts to become visible within 1 hour of death, and become more pronounced within 4 hours. Within the first 4 hours, lividity stains are not fixed and, if the body is moved, the blood products will shift and stain the part of the body that has become lower. In most cases, these stains become fixed between 12 and 24 hours. As such, they can be viewed as an indicator of how the body was left at the time of death. Importantly, if a body is found with post-mortem lividity stains not at the lowest point in the body, it can be concluded that the body has been moved or repositioned after the 12 to 24 hour stain setting period.9
Decomposition
This is the final indicator a pathologist can look at to estimate the time of death. Sometimes, dead bodies are not discovered in time to use body temperature, rigor mortis, or early lividity indicators to estimate a more exact time of death. In these cases, assessing the progress of decomposition becomes important. Decomposition starts as soon as the body ceases to be alive. Readable signs of decomposition will become apparent 36 to 48 hours after death.9 However, decompositional processes depend, to a large extent, on environmental factors, including temperature, humidity, light, rainfall, soil, location, the condition of the corpse,6 the time elapsed since burial, cause of death, whether the body was wrapped in cloth, plastic or other material, scavenging, post burial weathering and erosion and anthropogenic landscape modifications.7
pH
pH is defined as the negative logarithm of the hydrogen ion concentration [H+] and it is easily measured in pH units with a pH meter [49]. The amount of hydrogen ions in the soil and its pH are inversely proportional, affecting the solubility of minerals and nutrients.1
While bone is better preserved in slightly alkaline and neutral soils, human mummification can occur in both acidic and alkaline environments. Proof of that is the analysis conducted at the Oriental Cemetery (Figueira da Foz, Portugal) where mean values of soil pH varied between 8.20 and 8.24, and two bodies were completely mummified. Moreover, a study using adipose tissue from the abdominal region of pigs (Sus scrofa) aimed to correlate adipocere formation with pH. In controlled environments, samples were buried in damp loamy sand soils with different pH values (pH 2.4, pH 5.2, pH 8.5, and pH 12.6), and collected after a period of twelve months. The most suitable environments for adipocere formation were the ones with a pH between 5 and 9, with the highest alkaline setting significantly inhibiting decomposition and the formation of adipocere. Adipocere is the natural postmortem conversion of adipose tissue into a solid material made predominately of saturated fatty acids. A common effect of adipocere formation is the production of a surface shell which shields enclosed soft tissues from further decay.1
The presence of lime has also shown to alter soil pH. During an experiment, pigs (Sus scrofa) were buried with hydrated lime, quicklime, and without any type of lime as a control treatment. While the graves without lime remained the same, alkalinity increased in soils with carcasses covered with lime. Additionally, lime slowed cadaveric decomposition, although the carcasses were all skeletonized when exhumed. It is worth noting that lime has long been used in burials with the belief that it will accelerate decomposition.1
Clothing
The presence of clothing protecting against decomposition has been shown in experiments using piglets. Partially clothed piglets were lain out in open land. The head often decomposed considerably more quickly than the rest of the body which was largely covered by the clothing.5 Inorganic items will decompose at different rates, from weeks to hundreds of years, depending on what they are composed of cotton, silk, leather, metal, alloys, glass or plastic.5 Generally, granular soils tend to promote decomposition, whereas clay rich and organic soils may preserve human tissue over prolonged periods of time.7
Elemental Analysis
Chemical elements (e.g., alkali metals and heavy metals) are present in all ecosystems, being commonly analyzed in forensic studies given that their signature is highly specific to each geographic area.1
In a grave, groundwater can gain access to the buried body and expose it to exogenous agents with chemical effects. Given that tissue enzymes are inherently sensitive to heavy metal ions often present in groundwater, inactivity of the enzymatic decay processes by heavy metal ions can result in postmortem tissue preservation. There are, however, concerns arising that anthropogenic factors may be responsible for contaminating groundwaters due to leakage from burials. An investigation conducted on soil samples from a cemetery in Ohio (USA) from the mid-1800s showed high levels of arsenic—commonly used as a coffin wood preservative—and high levels of copper, iron, lead, and zinc used in the construction of coffins. Due to lixiviation, these elements can also contaminate groundwater.1
Decomposition Stages
A body passes through several stages as decomposition progresses driven by dehydration and discernible by characteristic gross taphonomic changes. The early stages of decomposition are wet and marked by discoloration of the flesh and the onset and cessation of bacterially-induced bloat. During early decay, intrinsic bacteria begin to digest the intestines from the inside out, eventually digesting away the surrounding tissues. Enzymes from within the dead cells of the cadaver also begin to break down tissues (autolysis). During putrefaction, bacteria undergo anaerobic respiration and produce gases as by-products such as hydrogen sulfide, methane, cadaverine, and putrescine. The buildup of resulting gas creates pressure, inflating the cadaver, and eventually forcing fluids out. This purging event marks the shift from early decomposition to late decomposition and may not be uniform; the head may purge before the trunk, for example. Purge may also last for some period of time in some parts of the body even as other parts of the body enter the most advanced stages of decomposition. In the trunk, purge is associated with an opening of the abdominal cavity to the environment. At this point, the rate of decay is reported by several authors to greatly increase as larval flies remove large portions of tissues; however, mummification may also occur, thus serving to preserve tissues. The final stages of decomposition last through to skeletonization and are the driest stages.4
In advanced stages of decomposition, the body is often no longer identifiable by facial recognition, and DNA testing or dental records become the tools to determine identity. At very advanced stages of decomposition, flies and maggots begin to emerge9 and can be used to estimate PMI.
Forensic Entomology
Insects are practically everywhere on Earth except the ocean and are closely associated with humans. Outdoor scenes are usually inhabited by numbers of flying insects, particularly adult flies, namely blowflies (Diptera: Calliphoridae), each waiting for specific habitat in a human environment to start a colony, as blooded or decomposing cadaver are deposited at the scene. This attracts surrounding flies and in a short timeframe their eggs are laid.10 Flies prefer injuries compared to natural body openings to lay their eggs (and for adult colonization).6
These eggs are then hatched and larval development begins after incubation and the development timeframe varies by species and ambient temperature at the scene.10 The number of life cycles of the maggot-to-fly can be estimated by a forensic entomologist to provide the amount of time that has passed since these insect life cycles began.9
Insects also use corpses as a breeding site, or they may predatorily feed on other corpse colonizers. Access for corpse-visiting insects to dead bodies is dependent on the environment. It is therefore not only the location where the corpse is found that must be considered (forest, town, field edge, buried, sunny, in shadow, indoors, and open land), but also the animals that are present there.6
The occurrence of beetles and beetle larvae is often associated with later stages of decomposition. Rove and dermestid beetles may, however, predatorily feed on fly maggots (rove) or actual corpse tissue (dermestids) earlier in succession. Several beetle species may occur during the entire decomposition process, even at unexpected times, due to climate change. The presence of adult beetles, their larvae, or the beetle’s feeding marks may provide evidence that the corpse was moved if the beetle species found on the corpse lives in restricted biotopes and is brought into a different biotope.6
There are only a few reports of wasps found on corpses. A study from Brazil reported wasps on dead pigs and the influence of wasps on the speed of decomposition. Wasps can feed on eggs of blow flies and thus wrongly reset the “developmental clock” on the postmortem interval. Certain species of wasp may prefer certain stages of decomposition. Their feeding activity causes traces on the body’s tissue similar to skin abrasions or scratch marks.6
TAPHONOMY: What Happened to the Remains After Death?
The term taphonomy was originally used to refer to the processes through which organic remains mineralize, also known as fossilization. Within the context of forensic anthropology, the term taphonomy is better defined as the study of what happens to human remains after death. Initial factors affecting a body after death include processes such as decomposition and scavenging by animals. However, taphonomic processes encompass much more than the initial period after death. For example, plant root growth can leach minerals from bone, leaving a distinctive mark. Sunlight can bleach human remains, leaving exposed areas whiter than those which remained buried. Water can wear the surface of the bone until it becomes smooth.2 Other Taphonomic forces include burial damage, fire damage, and mineral absorption.2
Some taphonomic processes can help a forensic anthropologist estimate the relative amount of time human remains have been exposed to the elements. For example, root growth through a bone would certainly indicate a body was buried for more than a few days. Forensic anthropologists must be very careful when attempting to estimate Postmortem Interval based on taphonomic processes as environmental conditions can greatly influence the rate at which taphonomic processes progress. For example, in cold environments, tissue may decay slower than in warm, moist environments.2
The study of the decomposition and placement of an individual’s body in the grave can also help bioarcheologists understand more about how the body was placed and treated. Corrosion of different kinds of metals placed as grave goods or used as material in coffins can stain bone. For example, in a sample of medieval and post-medieval Spanish and Basque skeletons from the Cathedral of Santa Maria, Kimberly Hopkinson and colleagues noted a bright green to turquoise staining in some of the skeletons’ teeth. The researchers believe that the staining was due to an ancient Greek practice of placing a coin in the mouth of the deceased to serve as the payment for the ferryman of Hades, Charon, who transported the deceased across the river that divided the world of the living from that of the dead. Hopkinson and colleagues determined that as the copper component of the bronze coins reacted with acid, it stained the teeth and surrounding bone, leaving evidence of the ancient Greek burial practice.2
Forensic anthropologists must contend with taphonomic processes that affect the preservation of bones. For example, high acidity in the soil can break down human bone to the point of crumbling. In addition, when noting trauma, they must be very careful not to confuse postmortem (after death) bone damage with trauma which occurs in the antemortem (before death) or perimortem (at or around the time of death) intervals.2
Scavengers
Land-based invertebrates may cause tissue defects (missing tissue) on deceased persons. Such wound artifacts, if they remain unnoticed, may cause misinterpretations and could be challenging during forensic case investigations. Feeding defects from birds, rodent, ants, and canines have also been reported.6 In addition to dogs and coyotes, the fox, opossum, vulture, raccoon, skunk, and crow are frequently involved in scattering and scavenging activity. Dog scavenging of neonates has been reported which features limb removal in contrast to patterns documented for larger individuals. Dog scavenging in indoor environments produces alterations to the face, neck, and arms in contrast to the pattern indicated in outdoor setting. Experiments have reported that when captive timber wolves (Canis lupus) feed on carcasses of deer (Odocoileus virginianus) the activity involves (1) movement of the carcass, opening of the thoracic cavity, and consumption of meaty sections and ribs; (2) disarticulation of one or more limbs and scattering of parts; and (3) destruction of long bone ends, the vertebral column, and rib ends. Dense bone ends and long bone diaphyses endured less damage.3
Rodents frequently contribute to scavenging of human remains. The porcupine, gerbil, mouse, squirrel, and rat are all known to transport and gnaw on bones leaving behind characteristic alterations. Bone gnawing activities have also been reported in the brown rat and gray squirrel. The woodland vole can produce gnaw marks in bone that are consistent with their incisor size.3
Raccoons represent frequent scavengers in geographic areas where they are abundant. Soft tissue are usually targeted by raccoons, especially from the arms and legs. The opossum also primarily concentrates on soft tissue but can relocate small bones short distances and can produce gnaw marks and punctures in small bones and on the margins of flat bones.3
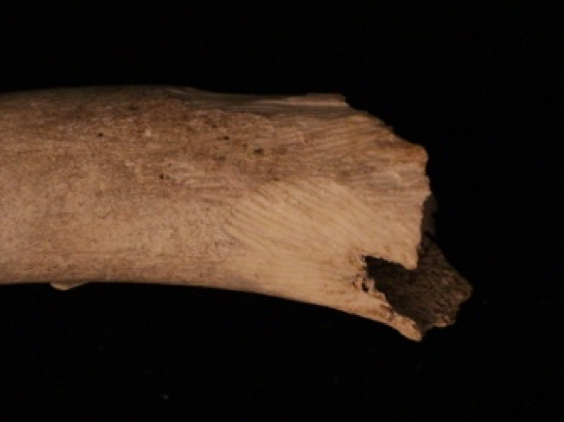
Rodent gnawing: When rodents, such as rats and mice, chew on bone, they leave sets of parallel grooves. The shallow grooves are etched by the rodent’s incisors (Figure 6.1).2
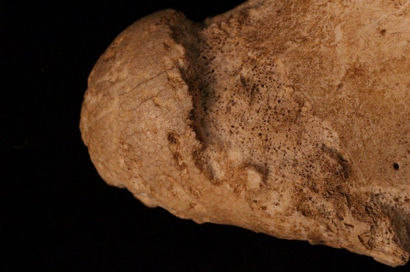
Carnivore damage: Like rodents, carnivores leave destructive dental marks on bone. Tooth marks may be visible be in form of pit marks or punctures from the canines, as well as extensive gnawing or chewing of the ends of the bones to retrieve marrow (Figure 6.2).2
In geographic regions where they live, vultures represent common scavengers of human remains. Their consumption of soft tissue can lead to rapid skeletonization. It has been noted that vultures can produce scratches on bone and often leave behind diagnostic feathers and feces.3
Although uncommon, cases involving shark scavenging have been reported. Shark activity is suggested by context, the pattern of injury, and recovery of fragments of shark teeth from the remains. A recent review of multiple cases involving apparent shark scavenging reports patterns of “incised gouges in cortical bone”, punctures, blunt force fractures, scratches, and fine incisions.3
Pigs are notorious scavengers but are rarely involved with human remains. However, when they do scavenge human remains, they leave characteristic tooth marks and concentration on the viscera, throat, and facial regions. Skeletal indications of pig scavenging include curvilinear scoring that differs from what is seen in carnivores or rodents due to differences in dental morphology, areas of missing cortical bone, and fragments of cortical bone displaced into the trabeculae.3
Bears are also infrequent scavengers of human remains. When bears are involved in scavenging activities, they create greater transport and removal of remains and scavenge for more prolonged periods than what is documented for smaller vertebrates. Bobcats have also been documented feeding on human remains producing soft tissue alterations and white-tailed deer have been pictured gnawing on human bone, producing superficial grooves in cortical bone.3
Fire Damage
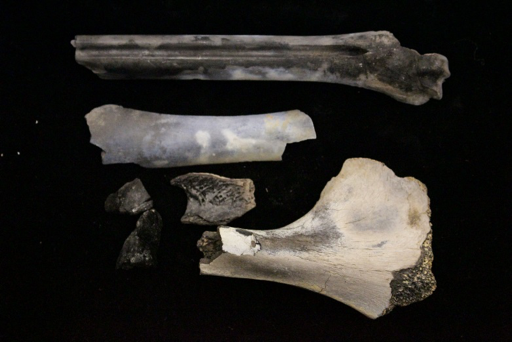
Burned bone: Fire causes observable damage to bone. Temperature and the amount of time bone is heated affect the appearance of the bone. Very high temperatures can crack bone and result in white coloration. Color gradients are visible in between high and lower temperatures, with lower temperatures resulting in black coloration from charring. Cracking can also reveal information about the directionality of the burn (Figure 6.3).2
Root Etching
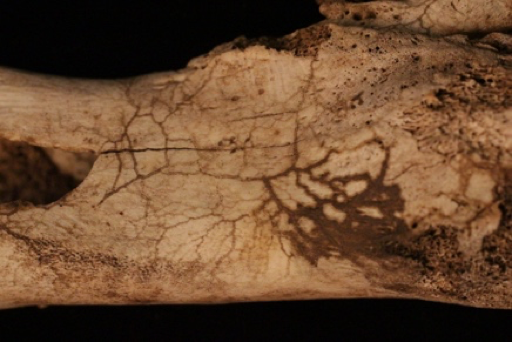
Root etching: Plants can alter bone. Specifically, plant roots can etch the outer surface of the bone, leaving grooves where the roots attached as they leached nutrients. During this process, the plant’s roots secrete acid that breaks down the surface of the bone (Figure 6.4).2
Weathering
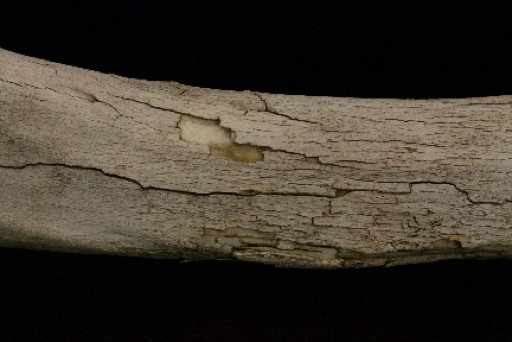
Weathering: Many different environmental conditions affect bone. River transport can smooth the surface of the bone due to water abrasion. Sunlight can bleach the exposed surface of bone. Dry and wet environments or the mixture of both types of environments can cause cracking and exfoliation of the surface. Burial in different types of soil can cause discoloration, and exposure can cause degreasing (Figure 6.5).2
Postmortem Alterations by Humans
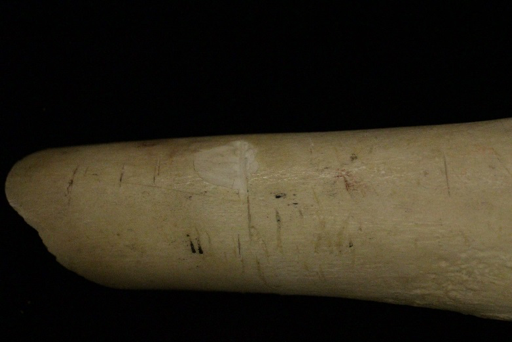
Cut marks: Humans also alter bone by cutting, scraping, or sawing it directly or in the process of removing tissue. The groove pattern—that is, the depth and width of the cuts—can help identify the tool used in the cutting process (Figure 6.6).2
References:
1. Angela Silva-Bessa, Áurea Madureira-Carvalho, Lorna Dawson, Maria Teresa Ferreira, Ricardo Jorge Dinis-Oliveira, and Shari L. Forbes, “The Importance of Soil on Human Taphonomy and Management of Portuguese Public Cemeteries,” Forensic Sciences 2 (2022): 635-649. https://www.mdpi.com/2673-6756/2/4/47.
2. Ashley Kendell, Alex Perrone, and Colleen Milligan, “Bioarcheology and Forensic Anthropology” In Explorations, ed. Beth Shook, Katie Nelson, Kelsie Aguilera and Lara Braff (Arlington: American Anthropological Association, 2019). https://pressbooksdev.oer.hawaii.edu/explorationsbioanth/chapter/osteology/.
3. Douglas H. Ubelaker and Cassandra M. DeGaglia, “The Impact of Scavenging: Perspective from Casework in Forensic Anthropology,” Forensic Sciences Research 5 (2020): 32–37. https://academic.oup.com/fsr/article/5/1/32/6794613.
4. Embriette R. Hyde, Daniel P. Haarmann, Aaron M. Lynne, Sibyl R. Bucheli , and Joseph F. Petrosino, “The Living Dead: Bacterial Community Structure of a Cadaver at the Onset and End of the Bloat Stage of Decomposition,” PLOs One (2013). https://journals.plos.org/plosone/article?id=10.1371/journal.pone.0077733.
5. J. Gordon Betts, Kelly A. Young, James A. Wise, Eddie Johnson, Brandon Poe, Dean H. Kruse, Oksana Korol, Jody E. Johnson, Mark Womble, Peter DeSaix, William Blaker, Julie Bowers, Matthew Barlow, Michael Hortsch, Emily Bradshaw, Kathleen Tallman, Boyd Campbell, Rohinton Tarapore, Branko Jablanovic, Pamela Dobbins, Brian Shmaefsky, Barbara Christie-Pope, Douglas Sizemore, Maurice Culver, Debra McLaughlin, Bruce Maring, Peter Dukehart, Susan Dentel, Elizabeth Tattersall, Margaret Weck, Neil Westergaard, Marnie Chapman, William Kleinelp, Brenda Leady, Susanna Heinze, Julie May, Ann Caplea, Kim Aaronson, Lopamudra Agarwal, Gary Allen, Nishi Bryska, Susan Caley Opsal, Norman Johnson, Sarah Leupen, Robert Mallet, Kenneth Crane, Heather Cushman, Chaya Gopalan, Mark Hubley, Dale Horeth, Kathleen Weiss, Sharon Ellerton, Janis Thompson, Rita Thrasher, Nicholas Mitchell, David Van Wylen, Elisabeth Martin, Ivan Paul, Lihua Liang, David Wortham, Shobhana Natarajan, Betsy Ott, Sondra Dubowsky, Mary Jane Niles, Mark Thomas, Mike Pyle, Umesh Yadav, Ann Henninger, Elizabeth DuPriest, Timothy Ballard, Phillip Nicotera, Michael Giangrande, Natalie Maxwell, Cameron Perkins, Robert Rawding, Lynn Gargan, Jeff Keyte, Victor Greco, Robert Allison, Heather Armbruster, Lynn Wandrey, David Pfeiffer, Mark Jonasson, Susan Spencer, Leigh Kleinert, Jason Schreer, Thomas Pilat, Ikemefuna Nwosu, Patty Dolan, Ellen DuPré, John Lepri, Carla Endres, Eileen Preston, Eric Sun, Tom Swenson, Tony Yates, Justin York, Cheri Zao, Elena Zoubina, Noelle Cutter, Lynnette Danzl-Tauer, Myriam Feldman, Jane Davis, Rosemary Hubbard, Aaron Payette, Greg Fitch, Robert Sullivan, AnnMarie DelliPizzi, Cynthia Standley, Shobhana Natarajan, Scott Payne, Laird Sheldahl, and Pam Elf, Anatomy and Physiology (Houston: Rice University, 2013). https://openstax.org/details/books/anatomy-and-physiology
6. Kristina Baumjohann and Mark Benecke, “Mind Your Decompositional Assumptions,” Forensic Sciences 2 (2022): 725-740. https://www.mdpi.com/2673-6756/2/4/54.
7. Laurance J. Donnelly and Mark Harrison, “Ground searches for graves and buried targets related to homicide, terrorism and organised crime,” Journal of International Geoscience 40 (2017). https://www.episodes.org/journal/view.html?volume=40&number=2&spage=106&vmd=A.
8. “Learning Modules,” eForenscis, accessed February 12, 2023, https://eforensics.info/learning_module/index/.
9. Rod Gehl and Darryl Plecas, Introduction to Criminal Investigation: Processes, Practices and Thinking (New Westminster, BC: Justice Institute of British Columbia, 2017). Download this book for free at https://pressbooks.bccampus.ca/criminalinvestigation/ as follows: digital format: on every electronic page print format: on at least one page near the front of the book.
10. Stephen J Morewitz, “Police determination of suspected foul play in death cases involving a body that is found at the death incident site: A case series,” Journal of Forensic Research 7 (2016): 71. https://www.hilarispublisher.com/conference-abstracts-files/2157-7145.C1.021_014.pdf.
Figure Attributions:
Figure 6.1 Rodent gnawing by Alex Perrone original to Explorations: An Open Invitation to Biological Anthropology is under a CC BY-NC 4.0 License.
Figure 6.2 Carnivore damage by Alex Perrone original to Explorations: An Open Invitation to Biological Anthropology is under a CC BY-NC 4.0 License.
Figure 6.3 Burned bone by Alex Perrone original to Explorations: An Open Invitation to Biological Anthropology is under a CC BY-NC 4.0 License.
Figure 6.4 Root etching by Alex Perrone original to Explorations: An Open Invitation to Biological Anthropology is under a CC BY-NC 4.0 License.
Figure 6.5 Weathering by Alex Perrone original to Explorations: An Open Invitation to Biological Anthropology is under a CC BY-NC 4.0 License.
Figure 6.6 Cut marks by Alex Perrone original to Explorations: An Open Invitation to Biological Anthropology is under a CC BY-NC 4.0 License.