6.8 Nutritional Adaptation
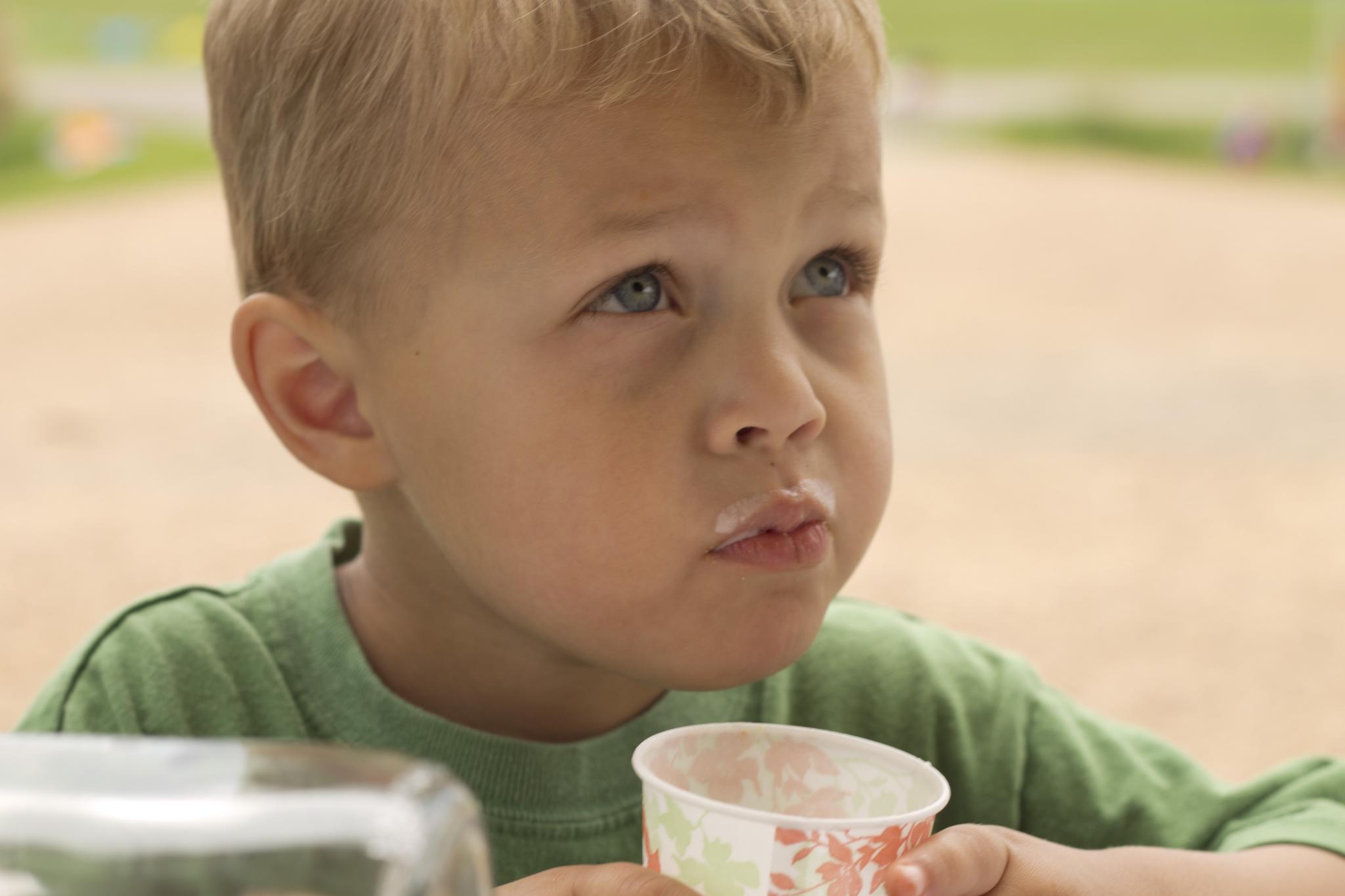
Got Lactase?
Do you remember the American “got milk?” slogan from the 1990s? It was used in advertisements for milk in which celebrities were pictured wearing milk “mustaches.” While the purpose of the “got milk?” ads was to sell more milk, there is no denying that drinking milk can actually be good for one’s health. Milk is naturally high in and minerals. It can also be low in fat or even fat-free if treated to remove the that naturally occur in milk. However, before you reach for a tall, cold glass of milk, you might want to ask yourself another question: “got lactase?”
Adaptation to Lactose
Do you drink milk? Or do you avoid drinking milk and consuming milk products because they cause you discomfort? If the latter is the case, then you may have trouble digesting milk.
Milk, Lactose, and Lactase
Milk naturally contains more than just proteins and lipids — it also contains carbohydrates. Specifically, milk contains the sugar lactose, which is a disaccharide (two-sugar) compound that consists of one molecule each of galactose and glucose, as shown in the structural formula below (Figure 6.8.2). Lactose makes up between two and eight per cent of milk by weight. The exact amount varies both within and between species.
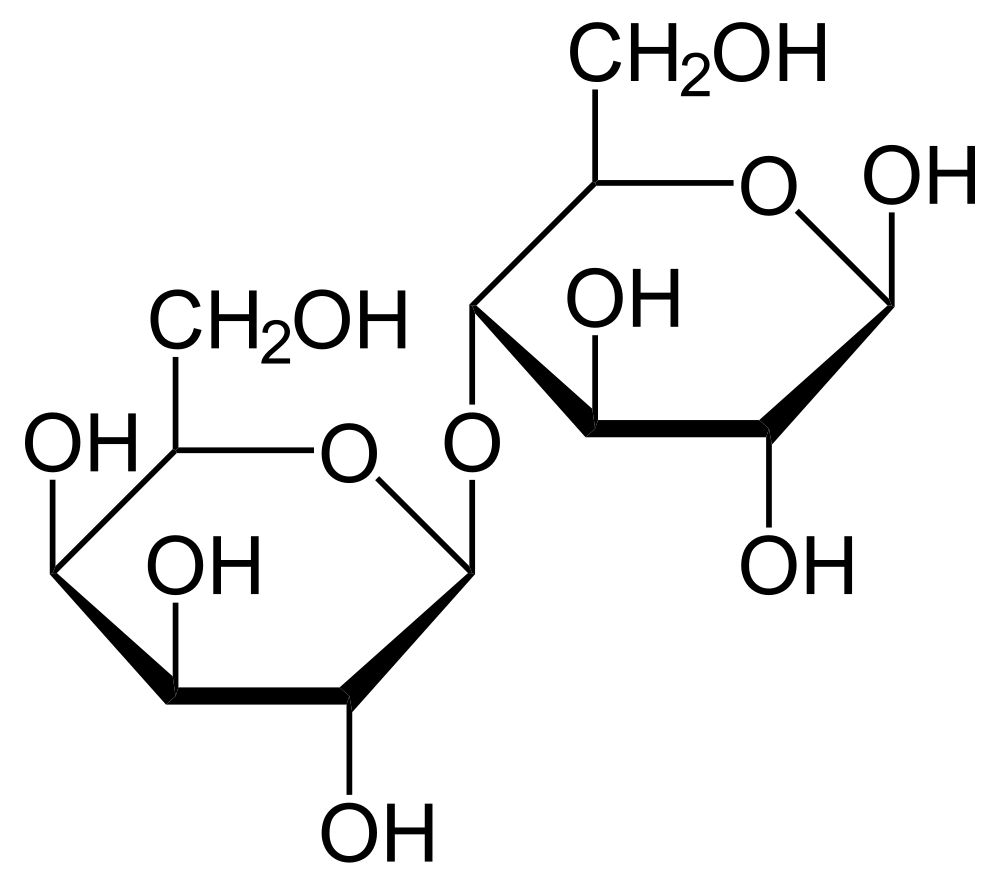
Lactose in milk must be broken down into its two component sugars to be absorbed by the small intestine. The enzyme lactase is needed for this process, as shown in Figure 6.8.3. Human infants are almost always born with the ability to synthesize lactase. This allows them to readily digest the lactose in their mother’s milk (or infant formula). In the majority of children, however, lactase synthesis begins to decline at about two years of age, and less and less lactase is produced throughout childhood.

Lactose Intolerance
Lactose intolerance is the inability of older children and adults to digest lactose in milk. People who are lactose intolerant may be able to drink small quantities of milk without any problems, but if they try to consume larger amounts, they are likely to suffer adverse effects. For example, they may have abdominal bloating and cramping, flatulence (gas), diarrhea, nausea, and vomiting. The symptoms may occur from 30 minutes to two hours after milk is consumed, and they’re generally worse when the quantity of milk consumed is greater. The symptoms result from the small intestine’s inability to digest and absorb lactose, so the lactose is passed on to the large intestine, where normal intestinal bacteria start breaking it down through the process of fermentation. This process releases gas and causes the other symptoms of lactose intolerance.
Lactose intolerance is actually the original and normal condition of the human species, as well as all other mammalian species. Early humans were hunter-gatherers that subsisted on wild plant and animal foods. The animal foods may have included meat and eggs, but did not include milk because animals had not been domesticated. Therefore, beyond the weaning period, milk was not available for people to drink in early human populations. It makes good biological sense to stop synthesizing an enzyme that the body does not need. After a young child is weaned, it is a waste of materials and energy to keep producing lactase when milk is no longer likely to be consumed.
Overall, an estimated 60 per cent of the world’s adult human population is thought to be lactose intolerant today. You can see the geographic distribution of modern human lactose intolerance on the map in Figure 6.8.4. Lactose intolerance (dark blue) approaches 100 per cent in populations throughout southern South America, southern Africa, and East and Southeast Asia.
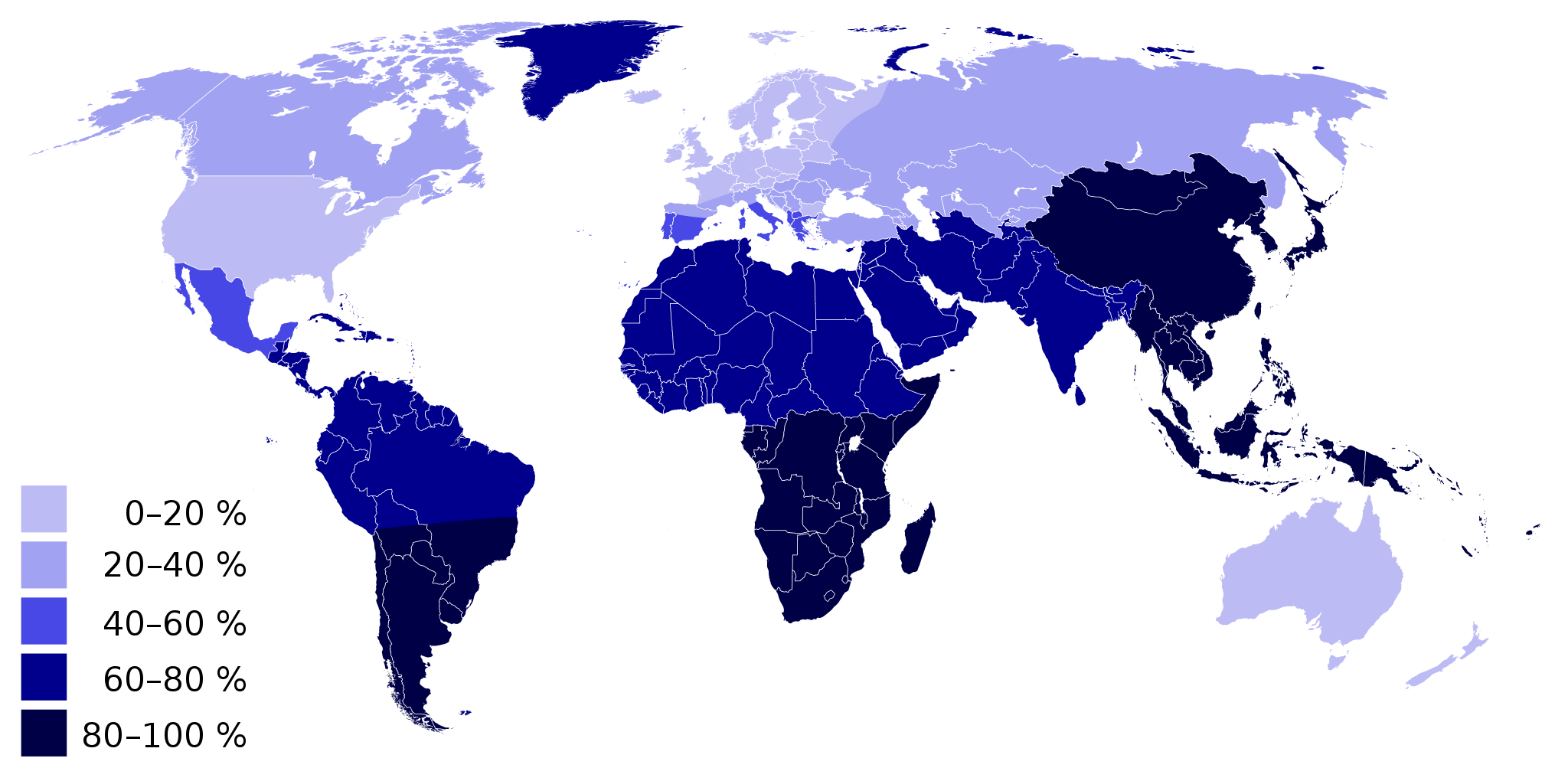
Lactose intolerance is not considered a medical problem, because its symptoms can be avoided by avoiding milk or milk products. Dietary control of lactose intolerance may be a matter of trial and error, however, because different people may be able to consume different quantities of milk or milk products before symptoms occur. If you are lactose intolerant, be aware that low-fat and fat-free milk may contain somewhat more lactose than full-fat milk because the former often have added milk solids that are relatively high in lactose.
Lactase Persistence
Lactase persistence is the opposite of lactose intolerance. People who are lactase persistent continue to produce the enzyme lactase beyond infancy and generally throughout life. As a consequence they are able to digest lactose and drink milk at older ages without adverse effects. The map in Figure 6.8.4 can also be read to show where lactase persistence occurs today. Populations with a low percentage of lactose intolerance (including most North Americans and Western and Northern Europeans) have high percentages of lactase-persistent people.
Lactase persistence is a uniquely human trait that is not found in any other mammalian species. Why did lactase persistence evolve in humans? When some human populations began domesticating and keeping herds of animals, animal milk became a potential source of food. Animals such as cows, sheep, goats, camels, and even reindeer (see Figure 6.8.5) can be kept for their milk. These animal milks also contain lactose, so natural selection would be strong for any individuals who kept producing lactase beyond infancy and could make use of this nutritious food. Eventually, the trait of lactase persistence would increase in frequency and come to be the predominant trait in dairying populations.
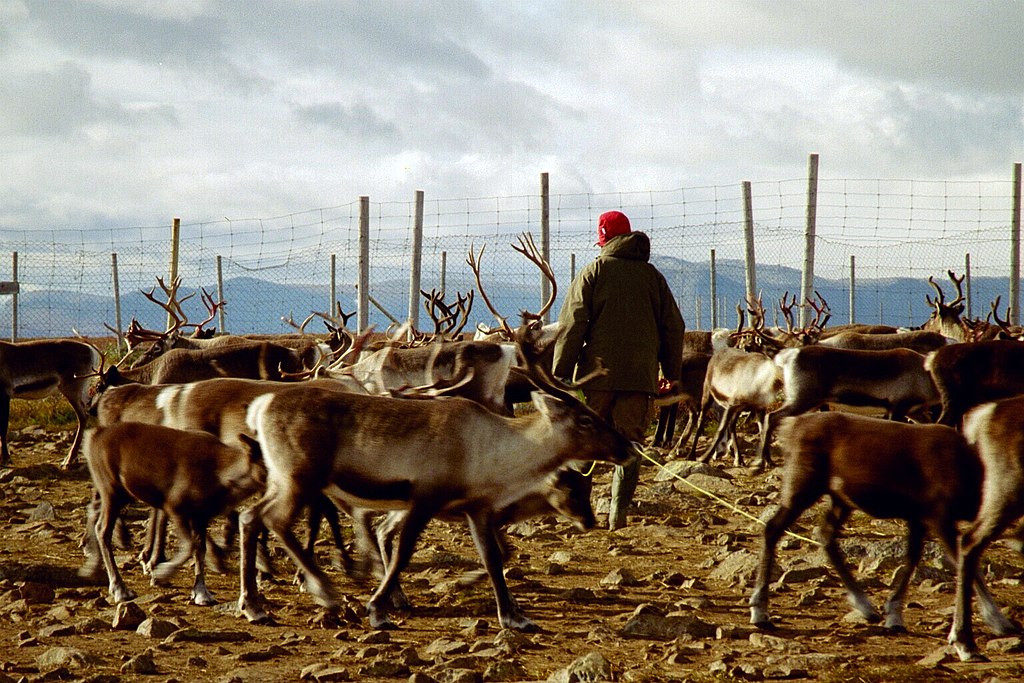
It is likely that lactase persistence occurs as a result of both genes and environment. Some people inherit genes that help them keep producing lactase after infancy. Geneticists think that several different mutations for lactase persistence arose independently in different populations within the last ten thousand years. Part of lactase persistence may be due to continued exposure to milk in the childhood and adulthood diet. In other words, a person may be genetically predisposed to synthesize lactase at older ages because of a mutation, but they may need the continued stimulation of milk drinking to keep producing lactase.
Thrifty Gene or Drifty Gene?
Besides variation in lactase persistence, human populations may vary in how efficiently they use calories in the foods they consume. People in some populations seem able to get by on quantities of food that would be inadequate for others, so they tend to gain weight easily. What explains these differences in people?
Thrifty Gene Hypothesis
In 1962, human geneticist James Neel proposed the . According to this hypothesis, so-called “thrifty genes” evolved in some human populations because they allowed people to get by on fewer calories and store the rest as body fat when food was plentiful. According to Neel’s hypothesis, thrifty genes would have increased in frequency through natural selection, because they would help people survive during times of famine. People with the genes would be fatter and able to rely on their stored body fat for calories when food was scarce.
Such thrifty genes would have been advantageous in early human populations of hunter-gatherers if food scarcity was a recurrent stress. However, in modern times, when most people have access to enough food year-round, thrifty genes would no longer be advantageous. In fact, under conditions of plentiful food, having thrifty genes would predispose people to gain weight and develop obesity. They would also tend to develop the chronic diseases associated with obesity, particularly type II diabetes. is a disease that occurs when there are problems with the pancreatic hormone insulin, which normally helps cells take up glucose from the blood and controls blood glucose levels. In type II diabetes, body cells become relatively resistant to insulin, leading to high blood glucose. This causes symptoms like excessive thirst and urination. Without treatment, diabetes can lead to serious consequences, such as blindness and kidney failure.
Neel proposed his thrifty gene hypothesis not on the basis of genetic evidence for thrifty genes, but as a possible answer to the mystery of why genes that seem to promote diabetes have not been naturally selected out of some populations. The mystery arose from observations that certain populations — such as South Pacific Islanders, sub-Saharan Africans, and southwestern Native Americans — developed high levels of obesity and diabetes after they abandoned traditional diets and adopted Western diets.
Assessing the Thrifty Gene Hypothesis
One of the assumptions underlying the thrifty gene hypothesis is that human populations that recently developed high rates of obesity and diabetes after Western contact had a long history of recurrent famine. Anthropological evidence contradicts this assumption for at least some of the populations in question. South Pacific Islanders, for example, have long lived in a “land of plenty,” with lush tropical forests year-round on islands surrounded by warm waters full of fish. Another assumption underlying the thrifty gene hypothesis is that hunter-gatherer people became significantly fatter during periods of plenty. Again, there is little or no evidence that hunter-gatherers traditionally deposited large fat stores when food was readily available.
Some geneticists have searched directly for so-called thrifty genes. Studies have revealed many genes with small effects associated with obesity or diabetes. However, these genes can explain only a few percentage points of the total population variation in obesity or diabetes.
The Drifty Gene and Other Hypotheses
Given the lack of evidence for the thrifty gene hypothesis, several researchers have suggested alternative hypotheses to explain population variation in obesity and diabetes. One hypothesis posits that susceptibility to obesity and diabetes may be a side effect of heat . According to this idea, some populations evolved lower metabolic rates as an adaptation to heat stress, because lower metabolic rates reduced the amount of heat that the body produced. The lower metabolic rates also predisposed people to gain excess weight and develop obesity and diabetes.
A thrifty phenotype hypothesis has also been proposed. This hypothesis suggests that individuals who have inadequate nutrition during fetal development might develop an insulin-resistant phenotype. The insulin-resistant phenotype would supposedly prepare these individuals for a life of famine, based on the environment within the womb. In a famine-free environment, however, the thrifty phenotype would lead to the development of diabetes.
The most recent alternative to the thrifty gene hypothesis is the drifty gene hypothesis, which was proposed by biologist John Speakman. He argues that genes protecting humans from obesity were under strong natural selection pressure for a very long period of time while human ancestors were subject to the risk of predation. According to this view, being able to outrun predators would have been an important factor selecting against fatness. When the risk of predation was lessened — perhaps as early as two million years ago — genes keeping fatness in check would no longer be selected for. Without selective pressure for these genes, their frequencies could change randomly due to genetic drift. In some populations, by chance, frequencies of the genes could decrease to relatively low levels, whereas in other populations the frequencies could be much higher.
Feature: Myth vs. Reality
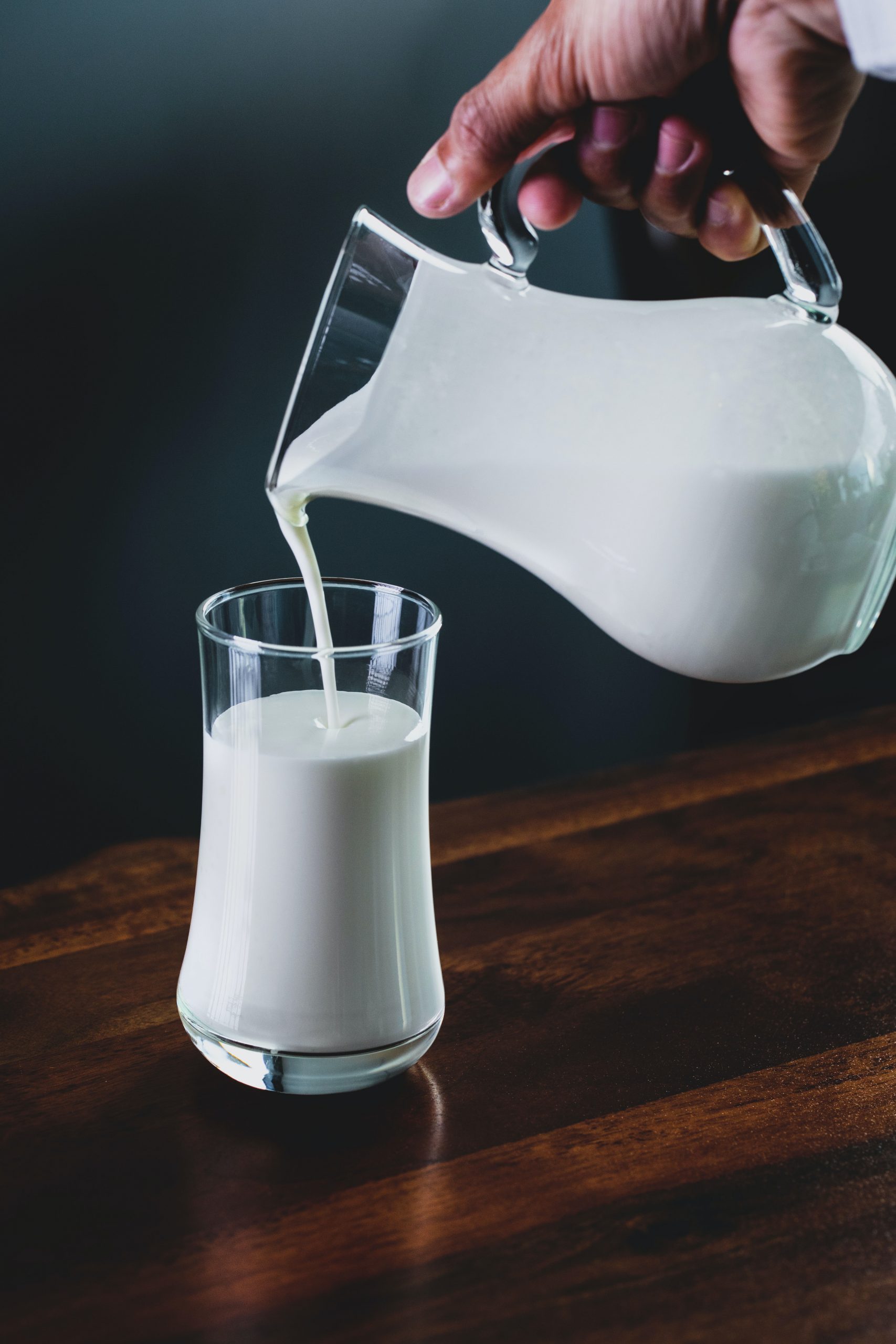
Myth: Lactose intolerance is an allergy to milk.
Reality: Lactose intolerance is not an allergy because it is not an immune system response. It is a sensitivity to milk caused by lactase deficiency so the sugar in milk cannot be digested. Milk allergy does exist, but it is a different condition that occurs in only about four per cent of people. It results when milk proteins (not milk sugar) trigger an immune reaction. How can you determine whether you have lactose intolerance or milk allergy? If you can drink lactose-free milk without symptoms, it is likely that you are lactose intolerant and not allergic to milk. However, if lactose-free milk also produces symptoms, it is likely that you have milk allergy. Note that it is possible to have both conditions.
Myth: If you are lactose intolerant, you will never be able to drink milk or consume other dairy products without suffering adverse physical symptoms.
Reality: Lactose intolerance does not mean that consuming milk and other dairy products is out of the question. Besides lactose-free milk, which is widely available, many dairy products have relatively low levels of lactose, so you may be able to consume at least small amounts of them without discomfort. You may be able to consume milk in the form of yogurt without any problems because the bacteria in yogurt produce lactase that breaks down the lactose. Greek yogurt may be your best bet, because it is lower in lactose to begin with. Aged cheeses also tend to have relatively low levels of lactose, because of the cheese-making process. Finally, by gradually adding milk or milk products to your diet, you may be able to increase your tolerance to lactose.
6.8 Summary
- Milk contains the sugar lactose, a disaccharide. Lactose must be broken down into its two component sugars to be absorbed by the small intestine, and the enzyme lactase is needed for this process.
- In about 60 per cent of people worldwide, the ability to synthesize lactase and digest lactose declines after the first two years of life. These people become lactose intolerant and cannot consume much milk without suffering symptoms of bloating, cramps, and diarrhea.
- In populations that herded milking animals for thousands of years, lactase persistence evolved. People who were able to synthesize lactase and digest lactose throughout life were strongly favored by natural selection. People — including many Europeans and European-Americans — who descended from these early herders generally still have lactase persistence.
- Human populations may vary in how efficiently they use calories in food. Some people (especially South Pacific Islanders, Native Americans, and sub-Saharan Africans) seem to be able to get by on fewer calories than would be adequate for others, so they tend to easily gain weight, become obese, and develop diseases such as diabetes.
- The thrifty gene hypothesis answers the question of how genes for this ability could have evolved. It proposes that “thrifty genes” were selected for because they allowed people to use calories efficiently and store body fat when food was plentiful so they had a reserve to use when food was scarce. Thrifty genes become detrimental and lead to obesity and diabetes when food is consistently plentiful.
- Several assumptions underlying the thrifty gene hypothesis have been called into question, and genetic research has been unable to actually identify thrifty genes. Alternate hypotheses to the thrifty gene hypothesis have been proposed, including the drifty gene hypothesis. The latter hypothesis explains variation in the tendency to become obese by genetic drift on neutral genes.
6.8 Review Questions
- Distinguish between the terms lactose and lactase.
- What is lactose intolerance, and what percentage of all people have it?
- Where and why did lactase persistence evolve?
- What is the thrifty gene hypothesis?
- How well is the thrifty gene hypothesis supported by evidence?
- Describe an alternative hypothesis to the thrifty gene hypothesis.
- Do you think that a lack of exposure to dairy products might affect a person’s lactase level? Why or why not?
- Describe an experiment you would want to do or data you would want to analyze that would help to test the thrifty phenotype hypothesis. Remember, you are studying people, so be sure it is ethical! Discuss possible confounding factors that you should control for, or that might affect the interpretation of your results.
- Explain the relationship between insulin, blood glucose, and type II diabetes.
6.8 Explore More
Why Are People Lactose Intolerant?, Super Scienced, 2016.
Peter Attia: What if we’re wrong about diabetes?, TED, 2013.
The Last Nomadic Reindeer Herders in the World, Great Big Story, 2018.
Experience a Traditional Whale Hunt in Northern Alaska | Short Film Showcase, National Geographic, 2018.
Attributions
Figure 6.8.1
IMG_4325 Milk Mustache licking 3 by Cedar Summit Farm on Flickr is used under a CC BY SA 2.0 (https://creativecommons.org/licenses/by-sa/2.0/) license.
Figure 6.8.2
Lactose Haworth by NEUROtiker on Wikimedia Commons is in the public domain (https://en.wikipedia.org/wiki/Public_domain).
Figure 6.8.3
Lactase by Boghog2 on Wikimedia Commons is released into the public domain (https://en.wikipedia.org/wiki/Public_domain).
Figure 6.8.4
Lactose Intolerance by Rainer Z … on Wikimedia Commons is released into the public domain (https://en.wikipedia.org/wiki/Public_domain).
Figure 6.8.5
Reindeer_herding by Mats Andersson on Wikimedia Commons is used under a CC BY 2.0 (https://creativecommons.org/licenses/by/2.0/deed.en) license.
Figure 6.8.6
Milk Photo [photo] by Eiliv-Sonas Aceron on Unsplash is used under the Unsplash License (https://unsplash.com/license).
References
Great Big Story. (2018, November 29). The last nomadic reindeer herders in the world. YouTube. https://www.youtube.com/watch?v=4O8k9qe8fjI&feature=youtu.be
Super Scienced. (2016, February 26). Why are people lactose intolerant? YouTube. https://www.youtube.com/watch?v=G1NGzycaQV0&feature=youtu.be
National Geographic. (2018, November 27). Experience a traditional whale hunt in northern Alaska | Short film showcase. YouTube. https://www.youtube.com/watch?v=XIYag5MWhPU&feature=youtu.be
TED. (2013, June 25). Peter Attia: What if we’re wrong about diabetes? YouTube. https://www.youtube.com/watch?v=UMhLBPPtlrY&feature=youtu.be
Wikipedia contributors. (2019, December 15). James V. Neel. In Wikipedia. https://en.wikipedia.org/w/index.php?title=James_V._Neel&oldid=930860629
Wikipedia contributors. (2020, June 9). John Speakman. In Wikipedia. https://en.wikipedia.org/w/index.php?title=John_Speakman&oldid=961610417
A class of biological molecule consisting of linked monomers of amino acids and which are the most versatile macromolecules in living systems and serve crucial functions in essentially all biological processes.
A substance that is insoluble in water. Examples include fats, oils and cholesterol. Lipids are made from monomers such as glycerol and fatty acids.
Created by: CK-12/Adapted by Christine Miller
Of Peas and People
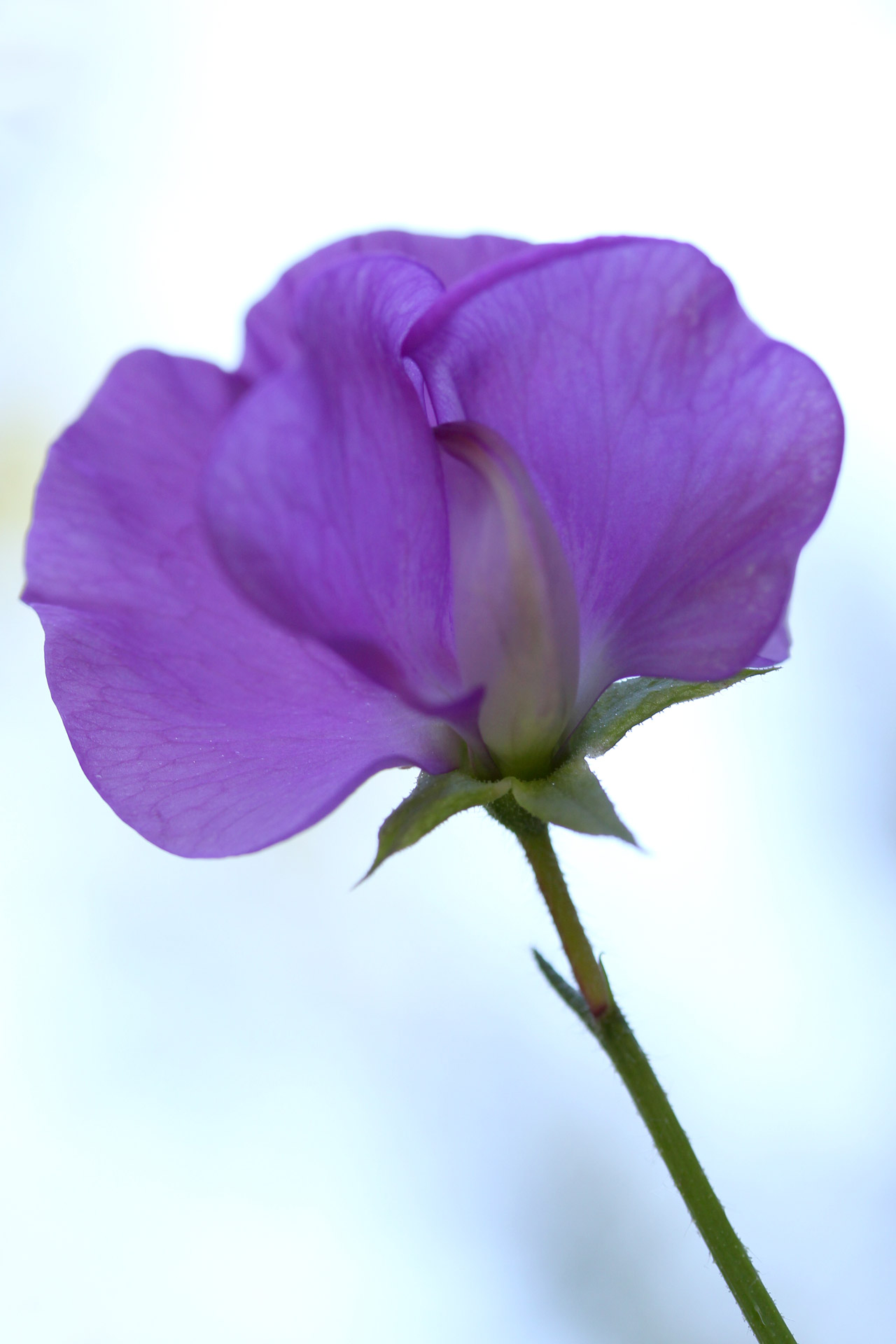
These purple-flowered plants are not just pretty to look at. Plants like these led to a huge leap forward in biology. They're common garden peas, and they were studied in the mid-1800s by an Austrian monk named Gregor Mendel. Through careful experimentation, Mendel uncovered the secrets of heredity, or how parents pass characteristics to their offspring. You may not care much about heredity in pea plants, but you probably care about your own heredity. Mendel's discoveries apply to people, as well as to peas — and to all other living things that reproduce sexually. In this concept, you will read about Mendel's experiments and the secrets of heredity that he discovered.
Mendel and His Pea Plants
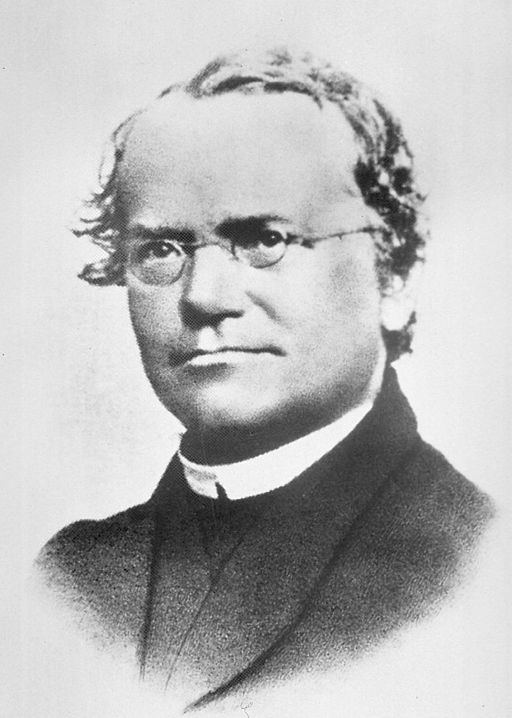
Gregor Mendel (Figure 5.10.2) was born in 1822. He grew up on his parents’ farm in Austria. He did well in school and became a friar (and later an abbot) at St. Thomas' Abbey. Through sponsorship from the monastery, he went on to the University of Vienna, where he studied science and math. His professors encouraged him to learn science through experimentation, and to use math to make sense of his results. Mendel is best known for his experiments with pea plants (like the purple flower pictured in Figure 5.10.1).
Blending Theory of Inheritance
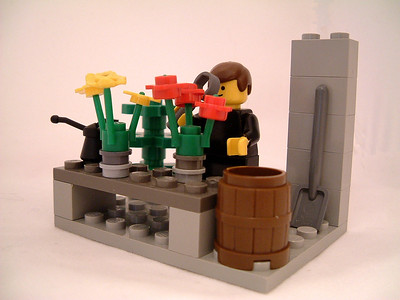
During Mendel's time, the blending theory of inheritance was popular. According to this theory, offspring have a blend (or mix) of their parents' characteristics. Mendel, however, noticed plants in his own garden that weren’t a blend of the parents. For example, a tall plant and a short plant had offspring that were either tall or short — not medium in height. Observations such as these led Mendel to question the blending theory. He wondered if there was a different underlying principle that could explain how characteristics are inherited. He decided to experiment with pea plants to find out. In fact, Mendel experimented with almost 30 thousand pea plants over the next several years!
Why Study Pea Plants?
Why did Mendel choose common, garden-variety pea plants for his experiments? Pea plants are a good choice because they are fast-growing and easy to raise. They also have several visible characteristics that can vary. These characteristics — some of which are illustrated in Figure 5.10.4 — include seed form and colour, flower colour, pod form and colour, placement of pods and flowers on stems, and stem length. Each of these characteristics has two common values. For example, seed form may be round or wrinkled, and flower colour may be white or purple (violet).
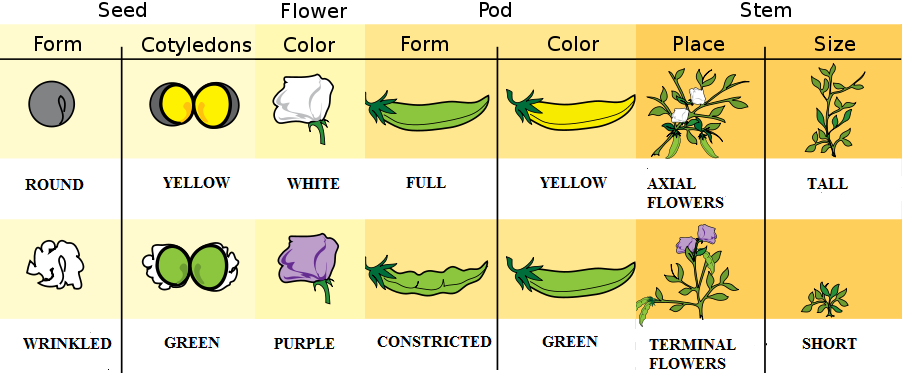
Controlling Pollination
To research how characteristics are passed from parents to offspring, Mendel needed to control , which is the fertilization step in the sexual reproduction of plants. Pollen consists of tiny grains that are the male sex cells (or gametes) of plants. They are produced by a male flower part called the anther. Pollination occurs when pollen is transferred from the anther to the stigma of the same or another flower. The stigma is a female part of a flower, and it passes pollen grains to female gametes in the ovary.
Pea plants are naturally self-pollinating. In , pollen grains from anthers on one plant are transferred to stigmas of flowers on the same plant. Mendel was interested in the offspring of two different parent plants, so he had to prevent self-pollination. He removed the anthers from the flowers of some of the plants in his experiments. Then he pollinated them by hand using a small paintbrush with pollen from other parent plants of his choice.
When pollen from one plant fertilizes another plant of the same species, it is called . The offspring that result from such a cross are called . When the term hybrid is used in this context, it refers to any offspring resulting from the breeding of two genetically distinct individuals.
Mendel's First Set of Experiments
At first, Mendel experimented with just one characteristic at a time. He began with flower colour. As shown in Figure 5.10.5, Mendel cross-pollinated purple- and white-flowered parent plants. The parent plants in the experiments are referred to as the P (for parent) generation.
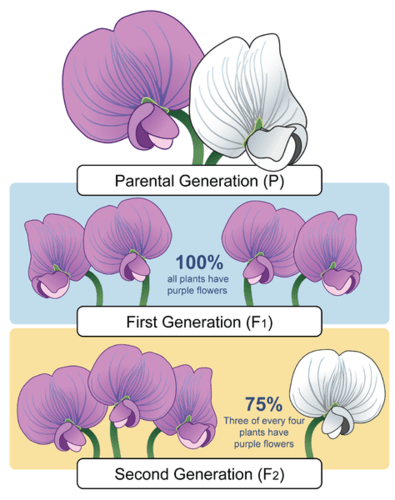
Figure 5.10.5 shows Mendel's first experiment with pea plants. The F1 generation results from the cross-pollination of two parent (P) plants, and it contains all purple flowers. The F2 generation results from the self-pollination of F1 plants, and contains 75% purple flowers and 25% white flowers.
F1 and F2 Generations
The offspring of the P generation are called the F1 (for filial, or “offspring”) generation. As shown in Figure 5.10.5, all of the plants in the F1 generation had purple flowers — none of them had white flowers. Mendel wondered what had happened to the white-flower characteristic. He assumed that some type of inherited factor produces white flowers and some other inherited factor produces purple flowers. Did the white-flower factor just disappear in the F1 generation? If so, then the offspring of the F1 generation — called the F2 generation — should all have purple flowers like their parents.
To test this prediction, Mendel allowed the F1 generation plants to self-pollinate. He was surprised by the results. Some of the F2 generation plants had white flowers. He studied hundreds of F2 generation plants, and for every three purple-flowered plants, there was an average of one white-flowered plant.
Law of Segregation
Mendel did the same experiment for all seven characteristics. In each case, one value of the characteristic disappeared in the F1 plants, later showing up again in the F2 plants. In each case, 75 per cent of F2 plants had one value of the characteristic, while 25 per cent had the other value. Based on these observations, Mendel formulated his first law of inheritance. This law is called the . It states that there are two factors controlling a given characteristic, one of which dominates the other, and these factors separate and go to different gametes when a parent reproduces.
Mendel's Second Set of Experiments
Mendel wondered whether different characteristics are inherited together. For example, are purple flowers and tall stems always inherited together, or do these two characteristics show up in different combinations in offspring? To answer these questions, Mendel next investigated two characteristics at a time. For example, he crossed plants with yellow round seeds and plants with green wrinkled seeds. The results of this cross are shown in Figure 5.10.6.
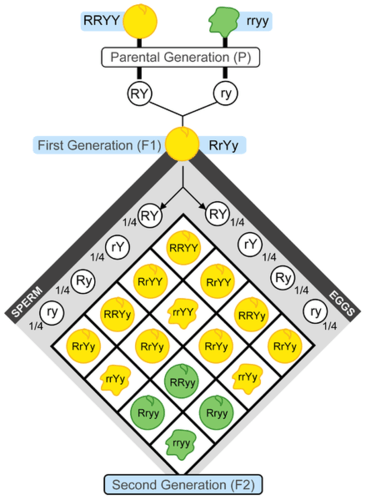
Figure 5.10.6 shows the outcome of a cross between plants that differ in seed colour (yellow or green) and seed form (shown here with a smooth round appearance or wrinkled appearance). The letters R, r, Y, and y represent genes for the characteristics Mendel was studying. Mendel didn’t know about genes, however, because genes would not be discovered until several decades later. This experiment demonstrates that, in the F2 generation, nine out of 16 were round yellow seeds, three out of 16 were wrinkled yellow seeds, three out of 16 were round green seeds, and one out of 16 was wrinkled green seeds.
F1 and F2 Generations
In this set of experiments, Mendel observed that plants in the F1 generation were all alike. All of them had yellow round seeds like one of the two parents. When the F1 generation plants self-pollinated, however, their offspring — the F2 generation — showed all possible combinations of the two characteristics. Some had green round seeds, for example, and some had yellow wrinkled seeds. These combinations of characteristics were not present in the F1 or P generations.
Law of Independent Assortment
Mendel repeated this experiment with other combinations of characteristics, such as flower colour and stem length. Each time, the results were the same as those shown in Figure 5.10.6. The results of Mendel's second set of experiments led to his second law. This is the . It states that factors controlling different characteristics are inherited independently of each other.
Mendel's Legacy
You might think that Mendel's discoveries would have made a big impact on science as soon as he made them, but you would be wrong. Why? Because Mendel's work was largely ignored. Mendel was far ahead of his time, and he was working from a remote monastery. He had no reputation in the scientific community and had only published sparingly in the past. Additionally, he published this research in an obscure scientific journal. As a result, when Charles Darwin published his landmark book on evolution in 1869, although Mendel's work had been published just a few years earlier, Darwin was unaware of it. Consequently, Darwin knew nothing about Mendel's laws, and didn’t understand heredity. This made Darwin's arguments about evolution less convincing to many.
Then, in 1900, three different European scientists — Hugo de DeVries, Carl Correns, and Erich von Tschermak — arrived independently at Mendel's laws. All three had done experiments similar to Mendel's and come to the same conclusions that he had drawn several decades earlier. Only then was Mendel's work rediscovered, so that Mendel himself could be given the credit he was due. Although Mendel knew nothing about genes, which were discovered after his death, he is now considered the father of genetics.
5.10 Cultural Connection
Corn is the world's most produced crop. Canada produces 13,000-14,000 metric Kilo tonnes of corn annually, mostly in fields in Ontario, Quebec and Manitoba. Approximately 1.5 million hectares are devoted to this crop which is critically important for both humans and livestock as a food source. Despite these high numbers of output, Canada is still only 11th on the list of world corn producers, with USA, China and Brazil claiming the top three places. How did corn become such an important part of modern agriculture?
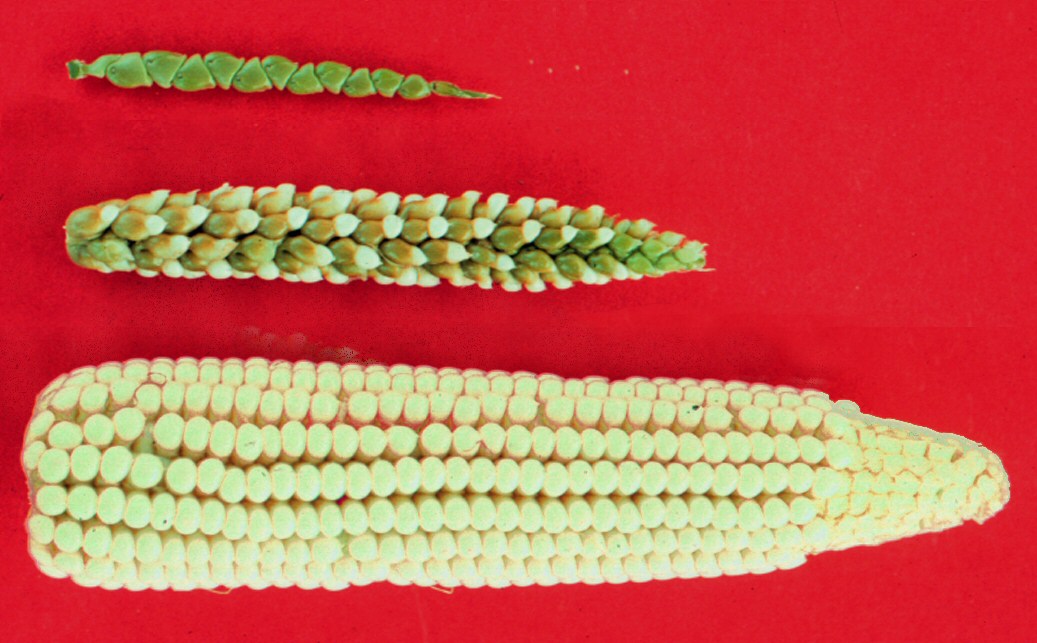
We didn't always have corn as we know it. Modern corn is descended from a type of grass called teosinte (Figure 5.10.7) native to Mesoamerica (southern part of North America). It is estimated that Indigenous people have been harvesting corn and corn ancestors for over 9000 years. Excavations of the Xihuatoxtla Shelter in southwestern Mexico revealed our earliest evidence of domesticated corn: maize remains on tools dating back 8,700 years.
Ancient Indigenous peoples of southern Mexico developed corn from grass plants using a process we now call , also known as . Teosinte doesn't resemble the corn we have today- it had only a few kernels individually encased on very hard shells, and yet today we have multiple varieties of corn with row upon row of bare kernels. This means that ancient agriculturalists among the Indigenous people of Mexico were intentionally cross-breeding strains of teosinte, and later, early maize to create plants which had more kernels, and reduced seed casings. Watch the TED Ed video in the Explore More section to see what other changes agriculturalists have made to modern-day corn.
5.10 Summary
- Mendel experimented with the inheritance of traits in pea plants at a time when the blending theory of inheritance was popular. This is the theory that offspring have a blend of the characteristics of their parents.
- Pea plants were good choices for this research, largely because they have several visible characteristics that exist in two different forms. By controlling pollination, Mendel was able to cross pea plants with different forms of the traits.
- In Mendel's first set of experiments, he experimented with just one characteristic at a time. The results of this set of experiments led to Mendel's first law of inheritance, called the . This law states that there are two factors controlling a given characteristic, one of which dominates the other, and these factors separate and go to different gametes when a parent reproduces.
- In Mendel's second set of experiments, he experimented with two characteristics at a time. The results of this set of experiments led to Mendel's second law of inheritance, called the . This law states that the factors controlling different characteristics are inherited independently of each other.
- Mendel's work was largely ignored during his own lifetime. However, when other researchers arrived at the same laws in 1900, Mendel's work was rediscovered, and he was given the credit he was due. He is now considered the father of genetics.
5.10 Review Questions
- Why were pea plants a good choice for Mendel's experiments?
- How did the outcome of Mendel's second set of experiments lead to his second law?
- Discuss the development of Mendel's legacy.
- If Mendel’s law of independent assortment was not correct, and characteristics were always inherited together, what types of offspring do you think would have been produced by crossing plants with yellow round seeds and green wrinkled seeds? Explain your answer.
5.10 Explore More
https://www.youtube.com/watch?v=Mehz7tCxjSE&feature=emb_logo
How Mendel's pea plants helped us understand genetics - Hortensia Jiménez Díaz, TED-Ed, 2013.
https://www.youtube.com/watch?v=ogc367xyzfk&feature=emb_logo
10 Strange Hybrid Fruits, Junkyboss, 2016.
https://www.youtube.com/watch?v=i6teBcfKpik
The history of the world according to corn - Chris A. Kniesly, TED-Ed, 2019.
Attributions
Figure 5.10.1
Purple sweet pea flower by unknown on Yana Ray on publicdomainpictures.net is used under a CC0 1.0 public domain dedication license (https://creativecommons.org/publicdomain/zero/1.0/deed.en).
Figure 5.10.2
Gregor_Mendel by unknown from National Institutes of Health, Health & Human Services on Wikimedia Commons is in the public domain (https://en.wikipedia.org/wiki/Public_domain).
Figure 5.10.3
Gregor Mendel in Lego by Alan on Flickr is used under a CC BY-NC-SA 2.0 (https://creativecommons.org/licenses/by-nc-sa/2.0/) license.
Figure 5.10.4
Mendels_peas by Mariana Ruiz [LadyofHats] on Wikimedia Commons is used under a CC0 1.0 public domain dedication license (https://creativecommons.org/publicdomain/zero/1.0/deed.en).
Figure 5.10.5
Mendel's first experiment with pea plants by CK-12 Foundation is used under a CC BY-NC 3.0 (https://creativecommons.org/licenses/by-nc/3.0/) license.
©CK-12 Foundation Licensed under
• Terms of Use • Attribution
Figure 5.10.6
Mendel's Second Experiment by by CK-12 Foundation is used under a CC BY-NC 3.0 (https://creativecommons.org/licenses/by-nc/3.0/) license.
©CK-12 Foundation Licensed under
• Terms of Use • Attribution
Figure 5.10.7
Maize-teosinte by John Doebley on Wikimedia Commons is used under a CC BY 3.0 (https://creativecommons.org/licenses/by/3.0/deed.en) license.
References
Brainard, J/ CK-12 Foundation. (2016). Figure 5 Mendel's first experiment [digital image]. In CK-12 College Human Biology (Section 5.9) [online Flexbook]. CK12.org. https://www.ck12.org/book/ck-12-human-biology/section/5.9/
Brainard, J/ CK-12 Foundation. (2016). Figure 6 Mendel's second experiment [digital image]. In CK-12 College Human Biology (Section 5.9) [online Flexbook]. CK12.org. https://www.ck12.org/book/ck-12-human-biology/section/5.9/
Junkyboss. (2016, March 31). 10 Strange hybrid fruits. YouTube. https://www.youtube.com/watch?v=ogc367xyzfk&feature=youtu.be
TED-Ed. (2013, March 12). How Mendel's pea plants helped us understand genetics - Hortensia Jiménez Díaz. YouTube. https://www.youtube.com/watch?v=Mehz7tCxjSE&feature=youtu.be
TED-Ed. (2019, November 26). The history of the world according to corn - Chris A. Kniesly. YouTube. https://www.youtube.com/watch?v=i6teBcfKpik&feature=youtu.be
Wikipedia contributors. (2020, June 1). Carl Correns. In Wikipedia. https://en.wikipedia.org/w/index.php?title=Carl_Correns&oldid=960172546
Wikipedia contributors. (2020, July 8). Charles Darwin. In Wikipedia. https://en.wikipedia.org/w/index.php?title=Charles_Darwin&oldid=966652322
Wikipedia contributors. (2020, March 9). Erich von Tschermak. In Wikipedia. https://en.wikipedia.org/w/index.php?title=Erich_von_Tschermak&oldid=944695823
Wikipedia contributors. (2020, July 7). Hugo de Vries. In Wikipedia. https://en.wikipedia.org/w/index.php?title=Hugo_de_Vries&oldid=966513671
Created by: CK-12/Adapted by Christine Miller
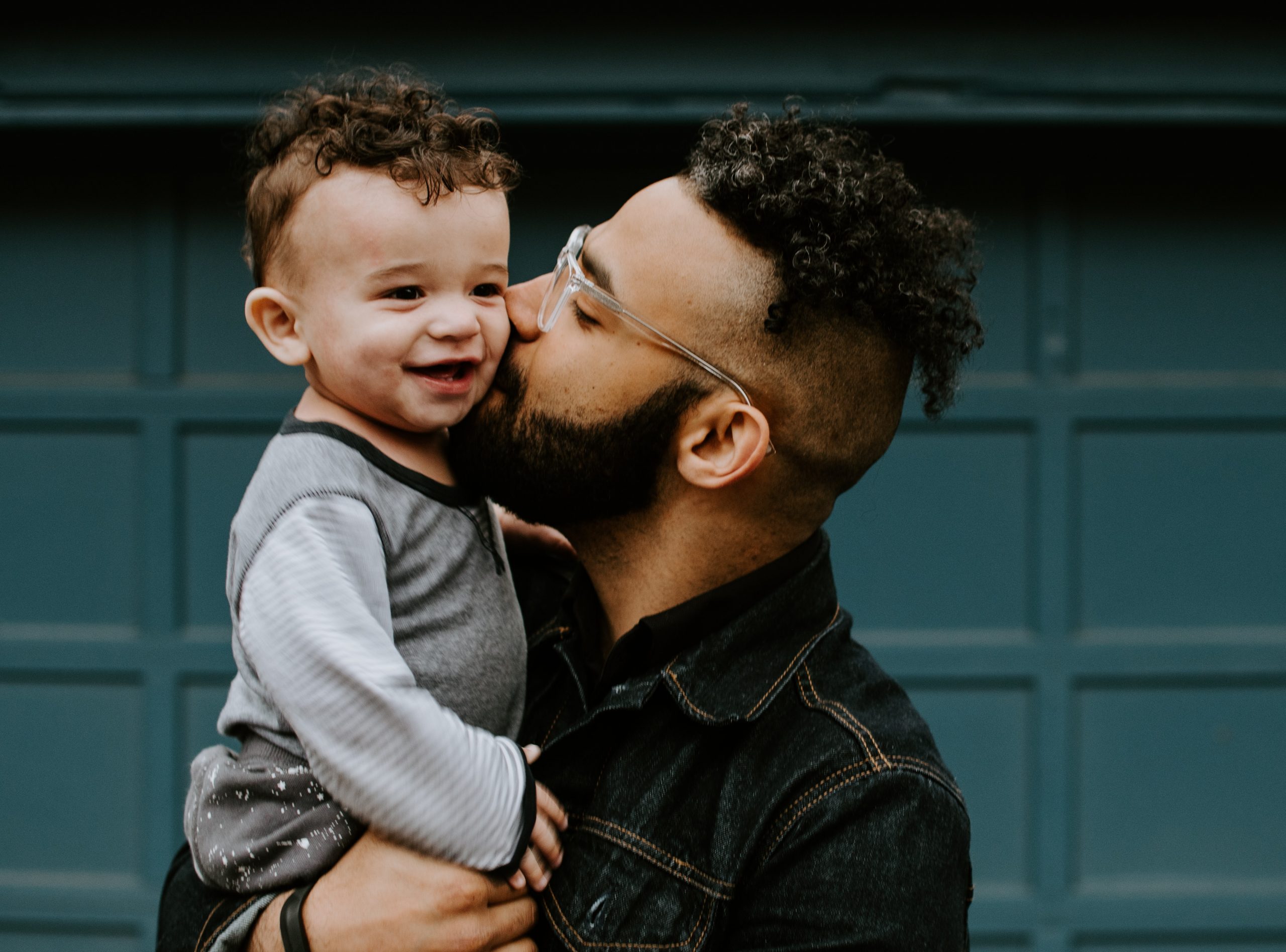
Like Father, Like Son
This father-son duo share some similarities. The shape of their faces and their facial features look very similar. If you saw them together, you might well guess that they are father and son. People have long known that the characteristics of living things are similar between parents and their offspring. However, it wasn’t until the experiments of Gregor Mendel that scientists understood how those traits are inherited.
The Father of Genetics
Mendel did experiments with pea plants to show how traits such as seed shape and flower colour are inherited. Based on his research, he developed his two well known laws of inheritance: the and the . When Mendel died in 1884, his work was still virtually unknown. In 1900, three other researchers working independently came to the same conclusions that Mendel had drawn almost half a century earlier. Only then was Mendel's work rediscovered.
Mendel knew nothing about genes, because they were discovered after his death. He did think, however, that some type of "factors" controlled traits, and that those "factors" were passed from parents to offspring. We now call these "factors" genes. , now expressed in terms of genes, form the basis of , the science of heredity. For this reason, Mendel is often called the father of genetics.
The Language of Genetics
Today, we know that traits of organisms are controlled by on . To talk about inheritance in terms of genes and chromosomes, you need to know the language of genetics. The terms below serve as a good starting point. They are illustrated in the figure that follows.
- A is the part of a that contains the genetic code for a given . For example, in pea plants, a given gene might code for flower colour.
- The position of a given gene on a chromosome is called its (plural, loci). A gene might be located near the center, or at one end or the other of a .
- A given gene may have different normal versions, which are called . For example, in pea plants, there is a purple-flower allele (B) and a white-flower allele (b) for the flower-colour gene. Different alleles account for much of the variation in the traits of organisms, including people.
- In sexually reproducing organisms, each individual has two copies of each type of chromosome. Paired chromosomes of the same type are called . They are about the same size and shape, and they have all the same genes at the same loci.
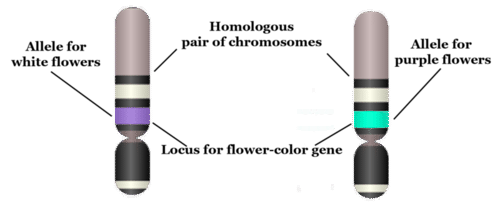
Genotype
When occurs, sex cells (called ) unite during to form a single cell called a . The zygote inherits two of each type of chromosome, with one chromosome of each type coming from the father, and the other coming from the mother. Because have the same genes at the same loci, each individual also inherits two copies of each gene. The two copies may be the same allele or different . The alleles an individual inherits for a given gene make up the individual’s . As shown in Table 5.11.1, an organism with two of the same allele (for example, BB or bb) is called a . An organism with two different alleles (in this example, Bb) is called a .
Table 5.11.1
Allele Combinations Associated With the Terms Homozygous and Heterozygous
Phenotype
The expression of an organism’s genotype is referred to as its , and it refers to the organism’s traits, such as purple or white flowers in pea plants. As you can see from Table 5.11.1, different genotypes may produce the same phenotype. In this example, both BB and Bb genotypes produce plants with the same phenotype, purple flowers. Why does this happen? In a Bb heterozygote, only the B allele is expressed, so the b allele doesn’t influence the phenotype. In general, when only one of two alleles is expressed in the phenotype, the expressed allele is called , and the allele that isn’t expressed is called .
The terms dominant and recessive may also be used to refer to phenotypic traits. For example, purple flower colour in pea plants is a dominant trait. It shows up in the phenotype whenever a plant inherits even one dominant allele for the trait. Similarly, white flower colour is a recessive trait. Like other recessive traits, it shows up in the phenotype only when a plant inherits two recessive alleles for the trait.
5.11 Summary
- Mendel's laws of inheritance, now expressed in terms of , form the basis of genetics, which is the science of heredity. This is why Mendel is often called the father of genetics.
- A gene is the part of a that codes for a given . The position of a gene on a chromosome is its . A given gene may have different versions, called . Paired chromosomes of the same type are called . They have the same size and shape, and they have the same genes at the same .
- The alleles an individual inherits for a given gene make up the individual's . An organism with two of the same allele is called a homozygote, and an individual with two different alleles is called a heterozygote.
- The expression of an organism's genotype is referred to as its . A dominant allele is always expressed in the phenotype, even when just one dominant allele has been inherited. A recessive allele is expressed in the phenotype only when two recessive alleles have been inherited.
5.11 Review Questions
- Define genetics.
- Why is Gregor Mendel called the father of genetics if genes were not discovered until after his death?
- Imagine that there are two alleles, R and r, for a given gene. R is dominant to r. Answer the following questions about this gene:
- What are the possible homozygous and heterozygous genotypes?
- Which genotype or genotypes express the dominant R phenotype? Explain your answer.
- Are R and r on different loci? Why or why not?
- Can R and r be on the same exact chromosome? Why or why not? If not, where are they located?
5.11 Explore More
https://www.youtube.com/watch?v=pv3Kj0UjiLE
Alleles and Genes, Amoeba Sisters, 2018.
https://www.youtube.com/watch?v=OaovnS7BAoc
Genotypes and Phenotypes, Bozeman Science, 2011.
Attributions
Figure 5.11.1
Father holding his baby boy with matching haircut [photo] by Kelly Sikkema on Unsplash is used under the Unsplash License (https://unsplash.com/license).
Figure 5.11.2
Chromosome, Gene, Locus, and Allele by CK-12 Foundation is used under a CC BY-NC 3.0 (https://creativecommons.org/licenses/by-nc/3.0/) license.
©CK-12 Foundation Licensed under
• Terms of Use • Attribution
Table 5.11.1
Allele Combinations Associated With the Terms Homozygous and Heterozygous by Christine Miller is released into the public domain (https://en.wikipedia.org/wiki/Public_domain).
References
Amoeba Sisters. (2018, February 1). Alleles and genes. YouTube. https://www.youtube.com/watch?v=pv3Kj0UjiLE&feature=youtu.be
Bozeman Science. (2011, August 4). Genotypes and phenotypes. YouTube. https://www.youtube.com/watch?v=OaovnS7BAoc&feature=youtu.be
Brainard, J/ CK-12 Foundation. (2016). Figure 2 Chromosome, gene, locus, and allele [digital image]. In CK-12 College Human Biology (Section 5.10) [online Flexbook]. CK12.org. https://www.ck12.org/book/ck-12-human-biology/section/5.9/
A genetically-based trait that has evolved because it helps living things survive and reproduce in a given environment.