7.3: ADAPTATION: INFECTIOUS DISEASE
Throughout our evolutionary journey, humans have been exposed to numerous infectious diseases. In the following section, we will explore some of the evolutionary-based adaptations that have occurred in certain populations in response to the stressors presented by select infectious diseases. One of the primary examples of natural selection processes acting on the human genome in response to the presence of an infectious disease is the case of the relationship between the sickle-cell anemia trait and malaria.
Malaria is a zoonotic disease (type of infectious disease naturally transmitted between animals and humans; covered in more detail in Chapter 16: Human Biology and Health) caused by the spread of the parasitic protozoa from the genus Plasmodium (Figure 7.1). These unicellular, eukaryotic protozoa are transmitted through the bite of a female Anopheles mosquito. During the bite process, the protozoan parasites that are present within an infected mosquito’s saliva will enter the bloodstream of the individual where they will be transported to the liver. Within the liver, the parasites multiply and will eventually be released into the bloodstream where they will infect erythrocytes. Once inside the erythrocytes, the parasites will reproduce until they exceed the cell’s storage capacity, causing it to burst and release the parasites into the bloodstream once again. This replication cycle will continue as long as there are viable erythrocytes within the host to infect.
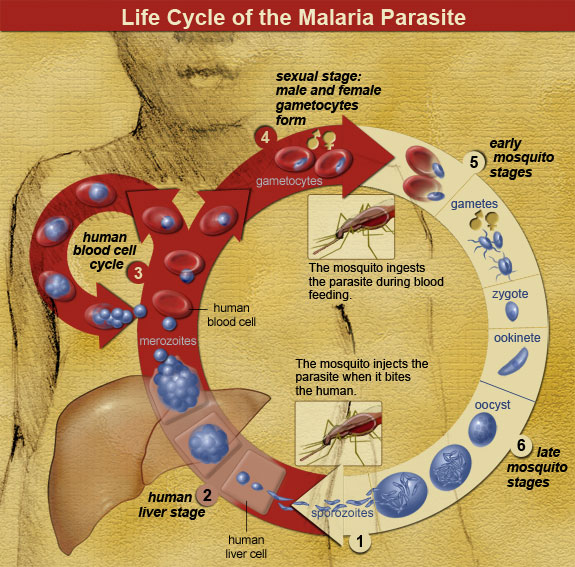
General complications from malaria infections include: enlargement of the spleen (due to destruction of infected erythrocytes), lower number of thrombocytes (also called platelets, required for coagulation/clotting of blood), high levels of bilirubin (a byproduct of hemoglobin breakdown in the liver) in the blood, jaundice (yellowing of the skin and eyes due to increased blood bilirubin levels), fever, vomiting, retinal (eye) damage, and convulsions (seizures). According to the World Health Organization, in 2016 there were 445,000 deaths from malaria globally with the highest percentage of those deaths occurring in Africa (91%) and Southeast Asia (6%) (World Health Organization 2017). In sub-Saharan Africa, where incidents of malaria are the highest in the world, 125 million pregnancies are affected by malaria, resulting in 200,000 infant deaths (Hartman et al. 2013). Pregnant women who become infected during the gestational process are more likely to have low-birthweight infants due to prematurity or growth restriction inside the uterus (Hartman et al. 2013). After birth, infants born to malaria-infected mothers are more likely to develop infantile anemia (low red blood cell counts), a malaria infection that is not related to the maternal malarial infection, and they are more likely to die than infants born to non-malaria-infected mothers (Hartman et al. 2013).
For children and adolescents whose brains are still developing, there is a risk of cognitive (intellectual) impairment associated with some forms of malaria infections (Fernando et al. 2010). Given the relatively high rates of morbidity (disease) and mortality (number of deaths) associated with malaria, it leads to reason that this disease may have served as a selective pressure during human evolution. Support for natural selection related to malaria resistance is related to genetic mutations associated with sickle cell, thalassemia, glucose-6-phosphate dehydrogenase (G6PD) deficiency, and the absence of certain antigens (molecules capable of inducing an immune response from the host) on erythrocytes. For the purposes of this text, we will focus our discussion on the relationship between sickle cell disease and malaria.
Sickle cell disease is a group of genetically inherited blood disorders characterized by an abnormality in the shape of the hemoglobin within erythrocytes. It is important to note that there are multiple variants of hemoglobin, including, but not limited to: A, D, C, E, F, H, S, Barts, Portland, Hope, Pisa, and Hopkins. Each of these variants of hemoglobin may result in various conditions within the body; however, for the following explanation we will focus solely on variants A and S.
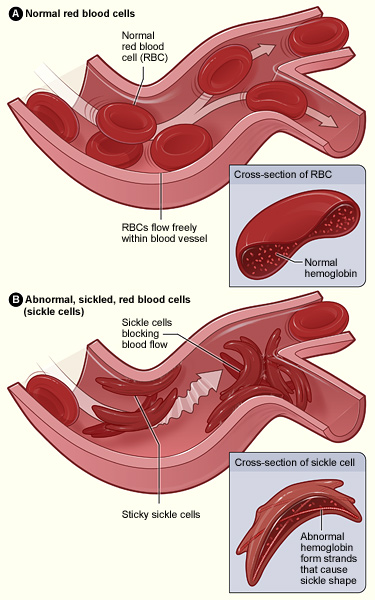
Individuals who inherit a mutated gene (hemoglobin with a sickled erythrocyte variety, HbS) on chromosome 11 from both parents will develop sickle cell anemia, which is the most severe form of the sickle cell disease family (Figure 7.2). The genotype of an individual with sickle cell anemia is HbSS; whereas, an individual without sickle cell alleles has a genotype of HbAA representing two normal adult hemoglobin type A variants. Manifestations of sickle cell anemia (HbSS) range from mild to severe with some of the more common symptoms being: anemia, blood clots, organ failure, chest pain, fever, and low blood oxygen levels. In high-income countries with advanced medical care, the median life expectancy of an HbSS individual is around 60 years; however, in low-income countries where advanced medical care is scarce, as many as 90% of children with sickle cell disease perish before the age of five (Longo et al. 2017).
Considering that advanced medical care was not available during much of human evolutionary history, it stands to reason that the majority of individuals with the HbSS genotype died before the age of reproduction. If that is the case though, why do we still have the HbS variant present in modern populations? As covered earlier in this textbook, the genotype of an individual is composed of genes from both biological parents. In the case of an individual with an HbSS genotype, the sickle cell allele (HbS) was inherited from each of the parents. For individuals with the heterozygous genotype of HbSA, they have inherited both a sickle cell allele (HbS) and a normal hemoglobin allele (HbA). Heterozygous (HbSA) individuals who reside in regions where malaria is endemic may have a selective advantage. They will experience a sickling of some, but not all, of their erythrocytes. Unlike an individual with the HbSS genotype, someone with HbSA may experience some of the symptoms listed above; however, they are generally less severe.
As noted earlier, the mechanism through which Plasmodium protozoan parasites replicate involves human erythrocyte cells. However, due to their sickled shape, as well as the presence of an abnormally shaped protein within the cell, the parasites are unable to replicate effectively in the erythrocyte cells coded for by the HbS allele (Cyrklaff et al. 2011). An individual who has an HbSA genotype and an active malaria infection will become ill with the disease to a lesser extent than someone with an HbAA genotype. Although normal erythrocytes (regulated by the HbA allele) allow for the replication of the parasite, the parasites will not be able to replicate in HbS erythrocytes of the heterozygote. So, individuals with the HbSA genotype are more likely to survive a malaria infection than an individual who is HbAA. Although individuals with the HbSA genotype may endure some physiological complications related to the sickling of some of their erythrocytes, their morbidity and mortality rates are lower than they are for HbSS members of the population. The majority of individuals who are heterozygous or homozygous for the HbS trait have ancestors who originated in sub-Saharan Africa, India, Saudi Arabia, regions in South and Central America, the Caribbean, and the Mediterranean (Turkey, Greece, and Italy) (Centers for Disease Control and Prevention 2017) (Figure 7.3).
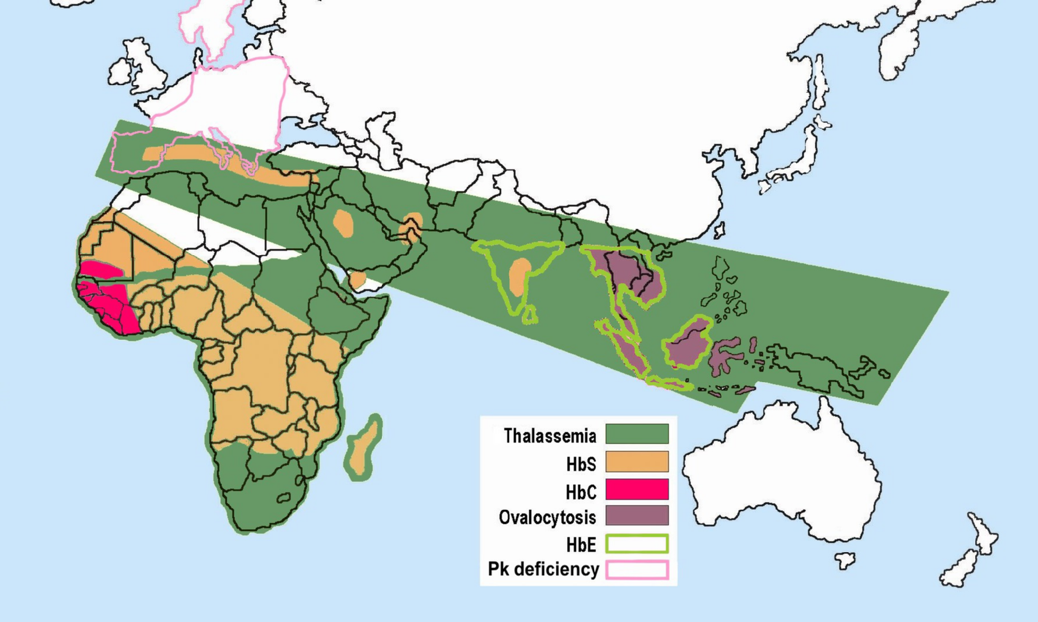
With respect to the history of these regions, during the early phases of settlement horticulture was the primary method of crop cultivation. Typically performed on a small scale, horticulture is based on manual labor and relatively simple hand tools rather than the use of draft animals or irrigation technologies. Common in horticulture is swidden, or the cutting and burning of plants in woodland and grassland regions. The swidden is the prepared field that results following a slash-and-burn episode. This practice fundamentally alters the soil chemistry, removes plants that provide shade, and increases the areas where water may pool. This anthropogenically altered landscape provides the perfect breeding ground for the Anopheles mosquito, as it prefers warm, stagnant pools of water (Figure 7.4).
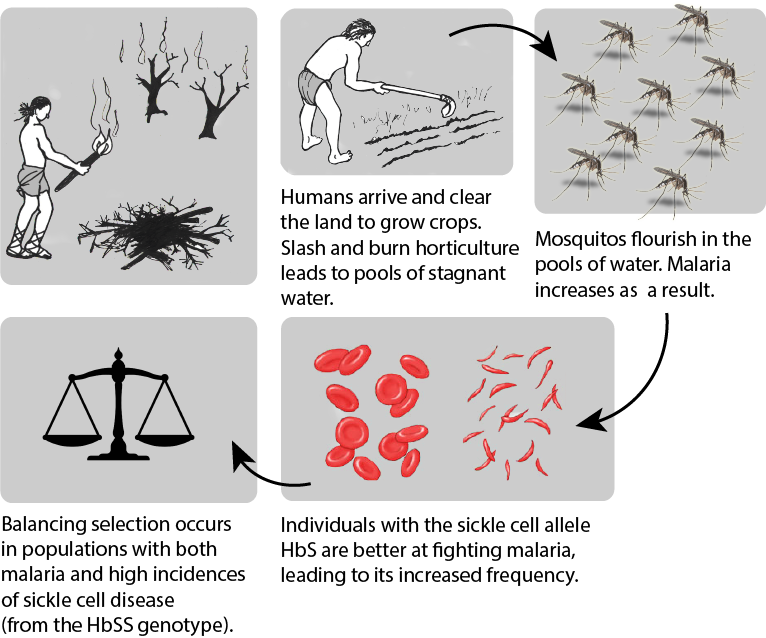
Although swidden agriculture was historically practiced across the globe, it became most problematic in the regions where the Anopheles mosquito is endemic. These areas have the highest incidence rates of malaria infection. Over time, the presence of the Anopheles mosquito and the Plasmodium parasite that it transmitted acted as a selective pressure, particularly in regions where swidden agricultural practices were common, toward the selection of individuals with some modicum of resistance against the infection. In these regions, HbSS and HbSA individuals would have been more likely to survive and reproduce successfully. Although individuals and populations are far more mobile now than they have been throughout much of history, there are still regions where we can see higher rates of malaria infection as well as greater numbers of individuals with the HbS erythrocyte variant. The relationship between malaria and the selective pressure for the HbS variant is one of the most prominent examples of natural selection in the human species within recent evolutionary history.
CITATION/ATTRIBUTION
Shook, B., Nelson, K., Aguilera, K., Braff, L., & Eds. (2019, December 9). Human Variation: An Adaptive Significance Approach. Pressbooks-Dev.oer.hawaii.edu. https://pressbooks-dev.oer.hawaii.edu/explorationsbioanth/chapter/__unknown__-13/