132 18.3 Sea-Floor Sediments — Physical Geology – 2nd Edition
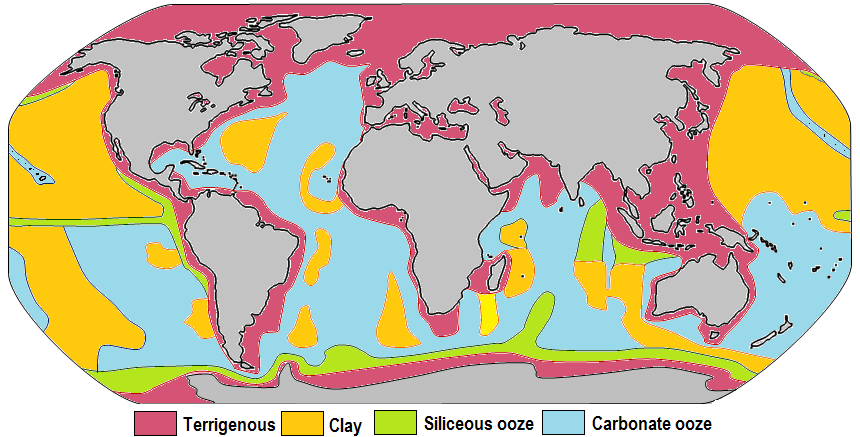
The distributions of some of these materials around the seas are shown in Figure 18.3.1. Terrigenous sediments predominate near the continents and within inland seas and large lakes. These sediments tend to be relatively coarse, typically containing sand and silt, but in some cases even pebbles and cobbles. Clay settles slowly in nearshore environments, but much of the clay is dispersed far from its source areas by ocean currents. Clay minerals are predominant over wide areas in the deepest parts of the ocean, and most of this clay is terrestrial in origin. Siliceous oozes (derived from radiolaria and diatoms) are common in the south polar region, along the equator in the Pacific, south of the Aleutian Islands, and within large parts of the Indian Ocean. Carbonate oozes are widely distributed in all of the oceans within equatorial and mid-latitude regions. In fact, clay settles everywhere in the oceans, but in areas where silica- and carbonate-producing organisms are prolific, they produce enough silica or carbonate sediment to dominate over clay.
Carbonate sediments are derived from a wide range of near-surface pelagic organisms that make their shells out of carbonate (Figure 18.3.2). These tiny shells, and the even tinier fragments that form when they break into pieces, settle slowly through the water column, but they don’t necessarily make it to the bottom. While calcite is insoluble in surface water, its solubility increases with depth (and pressure) and at around 4,000 metres, the carbonate fragments dissolve. This depth, which varies with latitude and water temperature, is known as the carbonate compensation depth, or CCD. As a result, carbonate oozes are absent from the deepest parts of the ocean (deeper than 4,000 metres), but they are common in shallower areas such as the mid-Atlantic ridge, the East Pacific Rise (west of South America), along the trend of the Hawaiian/Emperor Seamounts (in the northern Pacific), and on the tops of many isolated seamounts.
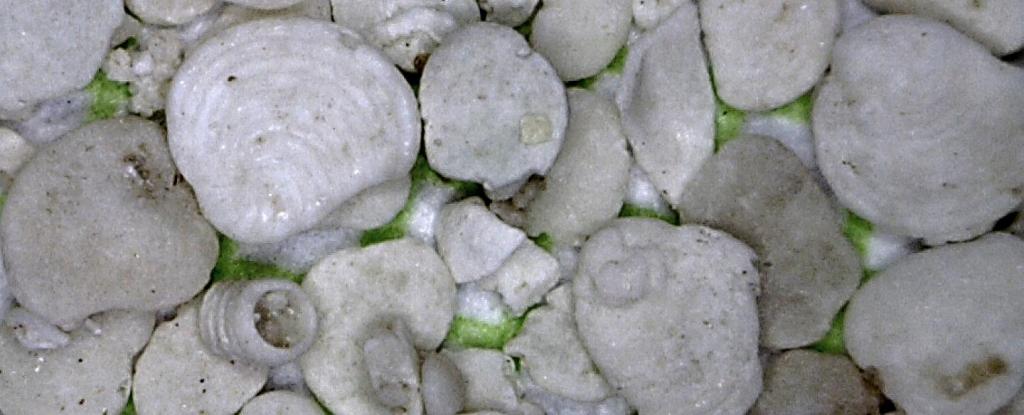
The diagram shows the sea floor in an area where there is abundant pelagic carbonate sediment. There is a continent within 100 kilometres of this area, to the right. What type of sediment (coarse terrigenous, clay, siliceous ooze, or carbonate ooze) would you expect at find at locations a, b, c, and d?
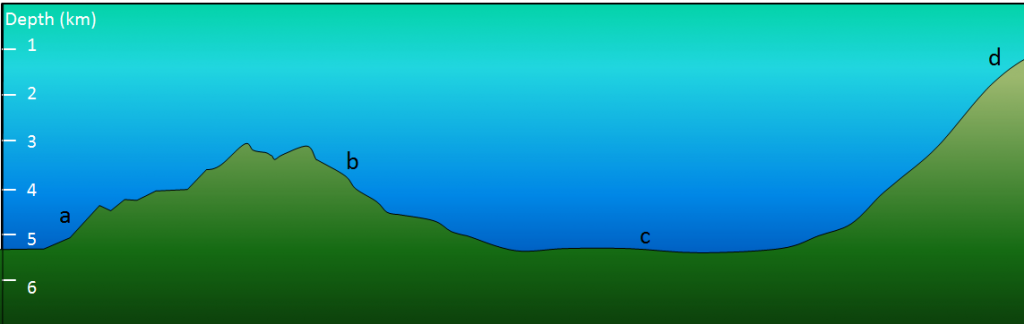
See Appendix 3 for Exercise 18.3 answers.
All terrestrial erosion products include a small proportion of organic matter derived mostly from terrestrial plants. Tiny fragments of this material plus other organic matter from marine plants and animals accumulate in terrigenous sediments, especially within a few hundred kilometres of shore. As the sediments pile up, the deeper parts start to warm up (from geothermal heat), and bacteria get to work breaking down the contained organic matter. Because this is happening in the absence of oxygen (a.k.a. anaerobic conditions), the by-product of this metabolism is the gas methane (CH4). Methane released by the bacteria slowly bubbles upward through the sediment toward the sea floor.
At water depths of 500 metres to 1,000 metres, and at the low temperatures typical of the sea floor (close to 4°C), water and methane combine to create a substance known as methane hydrate. Within a few metres to hundreds of metres of the sea floor, the temperature is low enough for methane hydrate to be stable and hydrates accumulate within the sediment (Figure 18.3.4). Methane hydrate is flammable because when it is heated, the methane is released as a gas (Figure 18.3.4). The methane within sea-floor sediments represents an enormous reservoir of fossil fuel energy. Although energy corporations and governments are anxious to develop ways to produce and sell this methane, anyone that understands the climate-change implications of its extraction and use can see that this would be folly. As we’ll see in the discussion of climate change in Chapter 19, sea-floor methane hydrates have had significant impacts on the climate in the distant past.
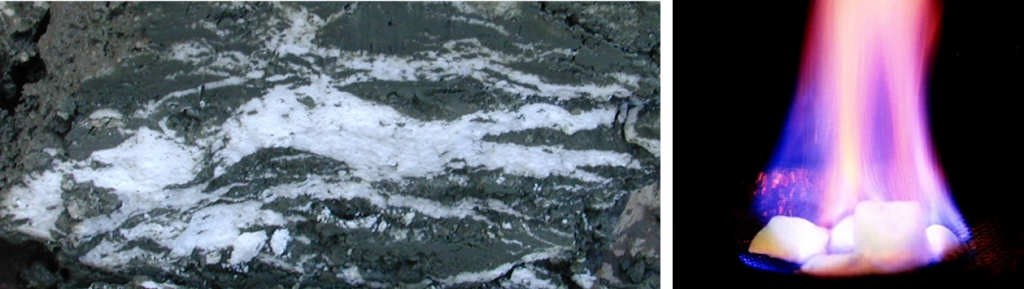
Image Descriptions
Figure 18.3.3 image description: A. is farthest from the continent. D is closest to the continent.
- A depth of 4.5 kilometres.
- A depth of 3.5 kilometres.
- A depth of 5 kilometres.
- A depth of 1 kilometre, close to the edge of a continent.
Media Attributions
- Figure 18.3.1, 18.3.2, 18.3.3: © Steven Earle. CC BY.
- Figure 18.3.4 (Left): “Gashydrat im Sediment” © Wusel007. CC BY-SA.
- Figure 18.3.4 (Right): “Burning Gas Hydrates” by J. Pinkston and L. Stern (USGS). Public domain.
<!– pb_fixme –>
<!– pb_fixme –>