79 10.4 Plate, Plate Motions, and Plate Boundary Processes — Physical Geology – 2nd Edition
10.4 Plate, Plate Motions, and Plate Boundary Processes
Continental drift and sea-floor spreading became widely accepted around 1965 as more and more geologists started thinking in these terms. By the end of 1967 the Earth’s surface had been mapped into a series of plates (Figure 10.4.1). The major plates are Eurasia, Pacific, India, Australia, North America, South America, Africa, and Antarctic. There are also numerous small plates (e.g., Juan de Fuca, Cocos, Nazca, Scotia, Philippine, Caribbean), and many very small plates or sub-plates. For example the Juan de Fuca Plate is actually three separate plates (Gorda, Juan de Fuca, and Explorer) that all move in the same general direction but at slightly different rates.
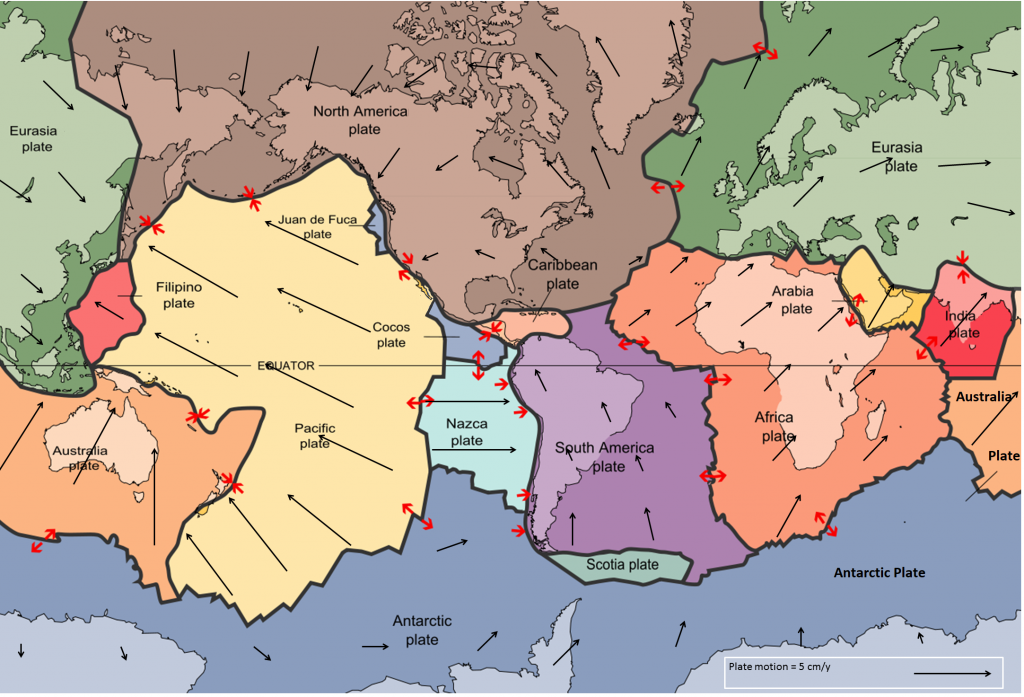
Rates of motions of the major plates range from less than 1 cm/y to over 10 cm/y. The Pacific Plate is the fastest, followed by the Australian and Nazca Plates. The North American Plate is one of the slowest, averaging around 1 cm/y in the south up to almost 4 cm/y in the north.
Plates move as rigid bodies, so it may seem surprising that the North American Plate can be moving at different rates in different places. The explanation is that plates move in a rotational manner. The North American Plate, for example, rotates counter-clockwise; the Eurasian Plate rotates clockwise.
Boundaries between the plates are of three types: divergent (i.e., moving apart), convergent (i.e., moving together), and transform (moving side by side). Before we talk about processes at plate boundaries, it’s important to point out that there are never gaps between plates. The plates are made up of crust and the lithospheric part of the mantle (Figure 10.4.2), and even though they are moving all the time, and in different directions, there is never a significant amount of space between them. Plates are thought to move along the lithosphere-asthenosphere boundary, as the asthenosphere is the zone of partial melting. It is assumed that the relative lack of strength of the partial melting zone facilitates the sliding of the lithospheric plates.
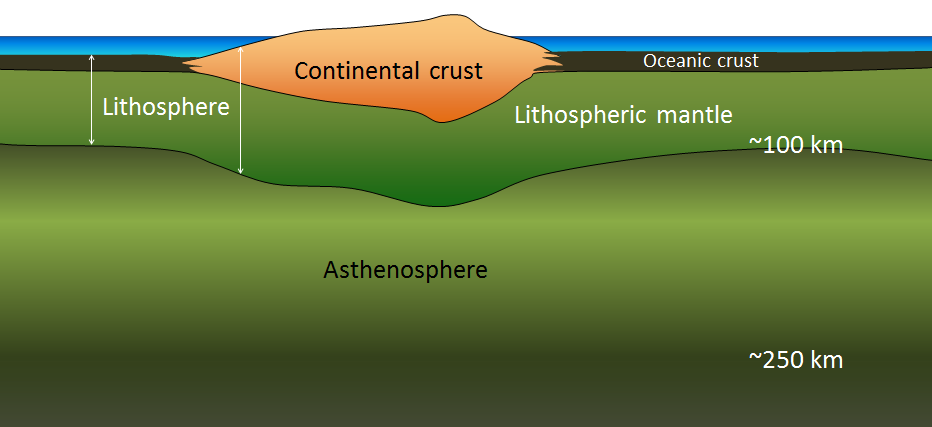
At spreading centers, the lithospheric mantle may be very thin because the upward convective motion of hot mantle material generates temperatures that are too high for the existence of a significant thickness of rigid lithosphere (Figure 10.3.8). The fact that the plates include both crustal material and lithospheric mantle material makes it possible for a single plate to be made up of both oceanic and continental crust. For example, the North American Plate includes most of North America, plus half of the northern Atlantic Ocean. Similarly the South American Plate extends across the western part of the southern Atlantic Ocean, while the European and African plates each include part of the eastern Atlantic Ocean. The Pacific Plate is almost entirely oceanic, but it does include the part of California west of the San Andreas Fault.
Divergent Boundaries
Divergent boundaries are spreading boundaries, where new oceanic crust is created from magma derived from partial melting of the mantle caused by decompression as hot mantle rock from depth is moved toward the surface (Figure 10.4.3). The triangular zone of partial melting near the ridge crest is approximately 60 km thick and the proportion of magma is about 10% of the rock volume, thus producing crust that is about 6 km thick. Most divergent boundaries are located at the oceanic ridges (although some are on land), and the crustal material created at a spreading boundary is always oceanic in character; in other words, it is mafic igneous rock (e.g., basalt or gabbro, rich in ferromagnesian minerals). Spreading rates vary considerably, from 2 cm/y to 6 cm/y in the Atlantic, to between 12 cm/y and 20 cm/y in the Pacific. (Note that spreading rates are typically double the velocities of the two plates moving away from a ridge.)
Some of the processes taking place in this setting include:
- Magma from the mantle pushing up to fill the voids left by divergence of the two plates
- Pillow lavas forming where magma is pushed out into seawater (Figure 10.4.4)
- Vertical sheeted dykes intruding into cracks resulting from the spreading
- Magma cooling more slowly in the lower part of the new crust and forming gabbro bodies
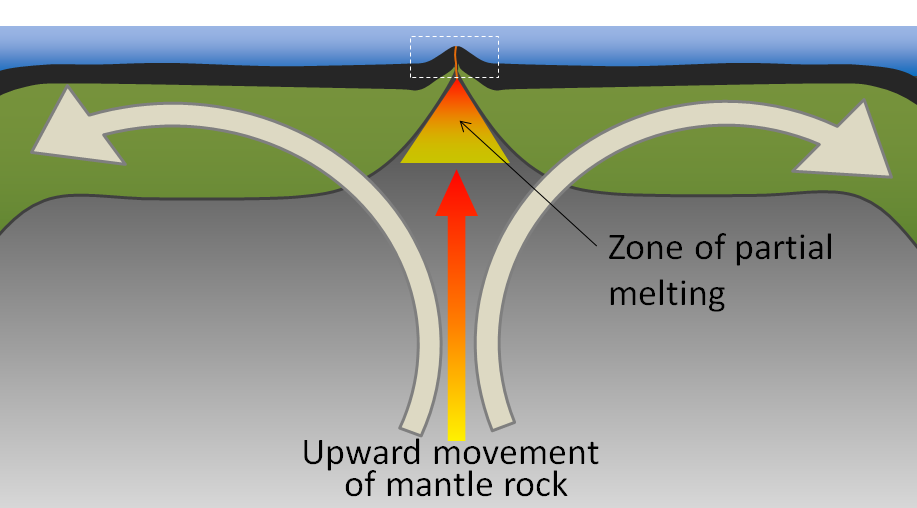
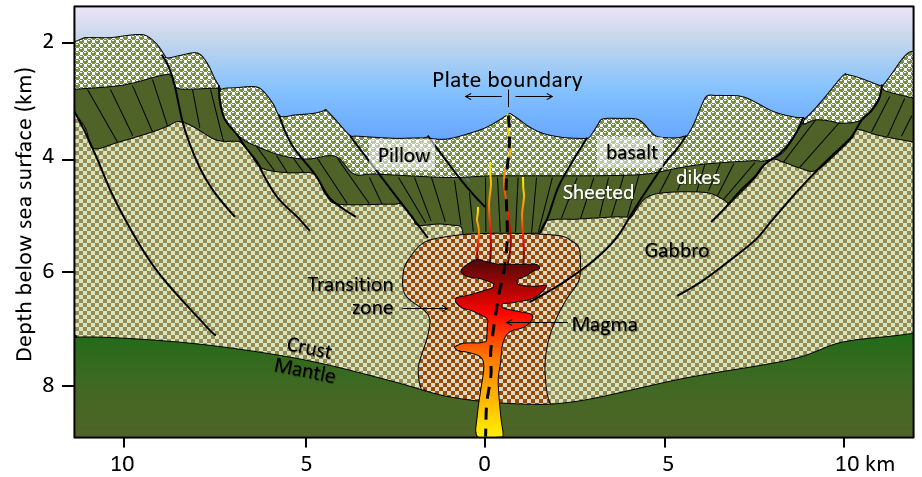
Spreading is hypothesized to start within a continental area with up-warping or doming related to an underlying mantle plume or series of mantle plumes. The buoyancy of the mantle plume material creates a dome within the crust, causing it to fracture in a radial pattern, with three arms spaced at approximately 120° (Figure 10.4.5). When a series of mantle plumes exists beneath a large continent, the resulting rifts may align and lead to the formation of a rift valley (such as the present-day Great Rift Valley in eastern Africa). It is suggested that this type of valley eventually develops into a linear sea (such as the present-day Red Sea), and finally into an ocean (such as the Atlantic). It is likely that as many as 20 mantle plumes, many of which still exist, were responsible for the initiation of the rifting of Pangea along what is now the mid-Atlantic ridge (see Figure 10.3.10).
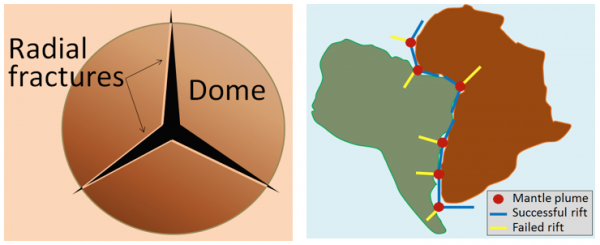
Convergent Boundaries
Convergent boundaries, where two plates are moving toward each other, are of three types, depending on whether oceanic or continental crust is present on either side of the boundary. The types are ocean-ocean, ocean-continent, and continent-continent.
At an ocean-ocean convergent boundary, one of the plates (oceanic crust and lithospheric mantle) is pushed, or subducted, under the other. Often it is the older and colder plate that is denser and subducts beneath the younger and hotter plate. There is commonly an ocean trench along the boundary. The subducted lithosphere descends into the hot mantle at a relatively shallow angle close to the subduction zone, but at steeper angles farther down (up to about 45°). As discussed in the context of subduction-related volcanism in Chapter 4, the significant volume of water within the subducting material is released as the subducting crust is heated. Most of this water is present within the sheet silicate mineral serpentine which is derived from alteration of pyroxene and olivine near the spreading ridge shortly after the rock’s formation. It is released when the oceanic crust is heats and then rises and mixes with the overlying mantle. The addition of water to the hot mantle lowers the rock’s melting point and leads to the formation of magma (flux melting) (Figure 10.4.6). The magma, which is lighter than the surrounding mantle material, rises through the mantle and the overlying oceanic crust to the ocean floor where it creates a chain of volcanic islands known as an island arc. A mature island arc develops into a chain of relatively large islands (such as Japan or Indonesia) as more and more volcanic material is extruded and sedimentary rocks accumulate around the islands.
As described above in the context of Benioff zones (Figure 10.3.6), earthquakes take place close to the boundary between the subducting crust and the overriding crust. The largest earthquakes occur near the surface where the subducting plate is still cold and strong.
Examples of ocean-ocean convergent zones are subduction of the Pacific Plate beneath the North America Plate south of Alaska (Aleutian Islands) and beneath the Philippine Plate west of the Philippines, subduction of the India Plate beneath the Eurasian Plate south of Indonesia, and subduction of the Atlantic Plate beneath the Caribbean Plate (see Figure 10.4.1).
At an ocean-continent convergent boundary, the oceanic plate is pushed under the continental plate in the same manner as at an ocean-ocean boundary. Sediment that has accumulated on the continental slope is thrust up into an accretionary wedge, and compression leads to thrusting within the continental plate (Figure 10.4.7). The mafic magma produced adjacent to the subduction zone rises to the base of the continental crust and leads to partial melting of the crustal rock. The resulting magma ascends through the crust, producing a mountain chain with many volcanoes.
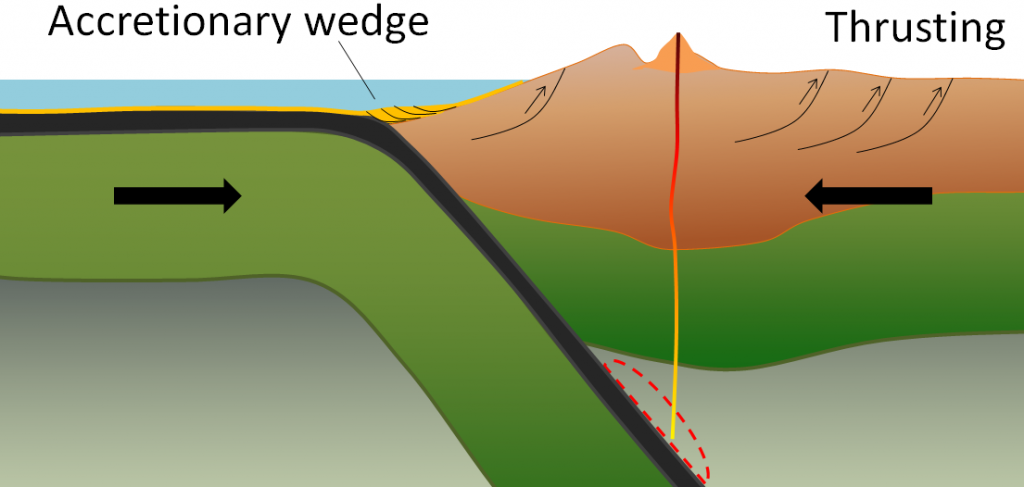
Examples of ocean-continent convergent boundaries are subduction of the Nazca Plate under South America (which has created the Andes Range) and subduction of the Juan de Fuca Plate under North America (creating the mountains Garibaldi, Baker, St. Helens, Rainier, Hood, and Shasta, collectively known as the Cascade Range).
A continent-continent collision occurs when a continent or large island that has been moved along with subducting oceanic crust collides with another continent (Figure 10.4.8). The colliding continental material will not be subducted because it is too light (i.e., because it is composed largely of light continental rocks [SIAL]), but the root of the oceanic plate will eventually break off and sink into the mantle. There is tremendous deformation of the pre-existing continental rocks, and creation of mountains from that rock, from any sediments that had accumulated along the shores (i.e., within geosynclines) of both continental masses, and commonly also from some ocean crust and upper mantle material.
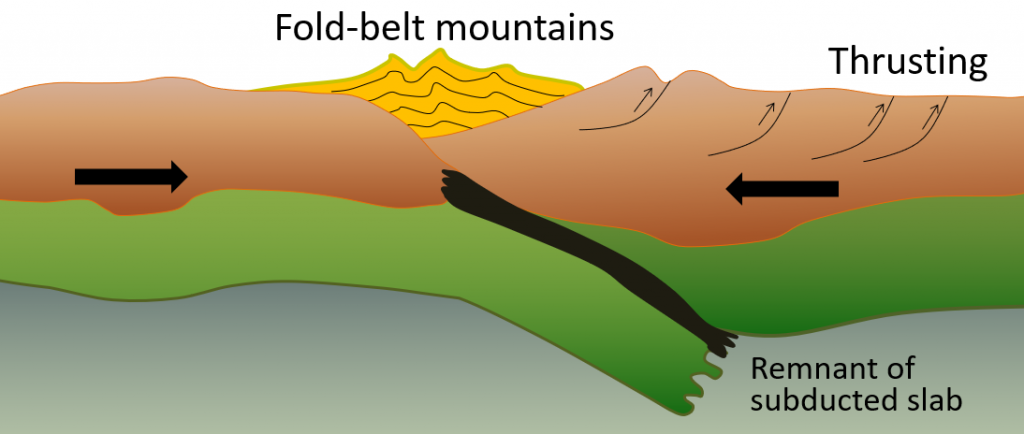
Examples of continent-continent convergent boundaries are the collision of the India Plate with the Eurasian Plate, creating the Himalaya Mountains, and the collision of the African Plate with the Eurasian Plate, creating the series of ranges extending from the Alps in Europe to the Zagros Mountains in Iran. The Rocky Mountains in B.C. and Alberta are also a result of continent-continent collisions.
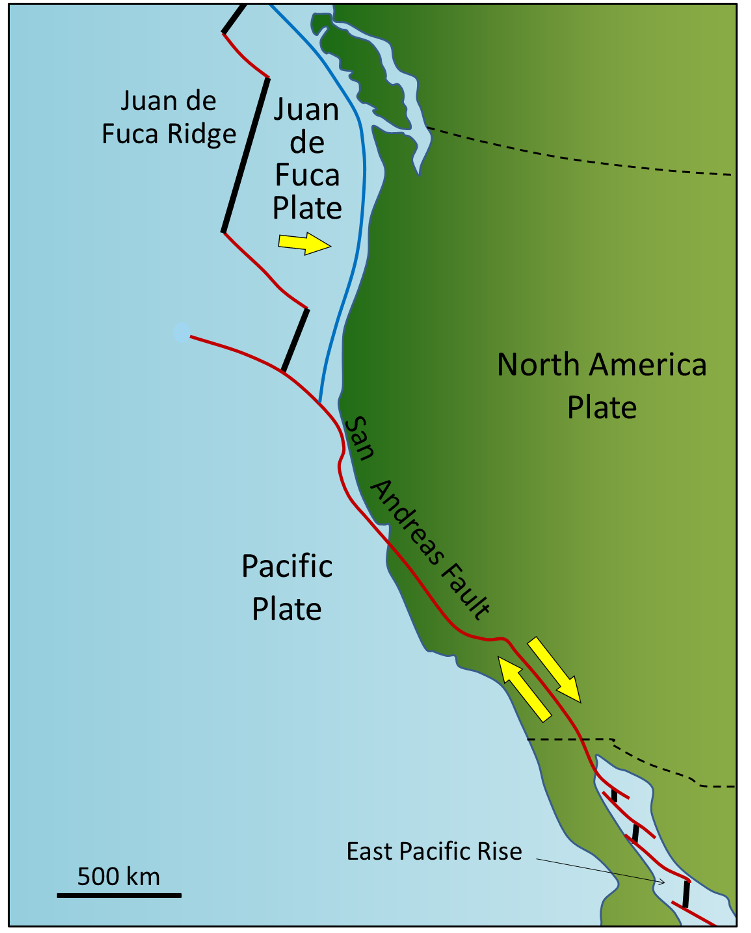
Transform boundaries exist where one plate slides past another without production or destruction of crustal material. As explained above, most transform faults connect segments of mid-ocean ridges and are thus ocean-ocean plate boundaries (Figure 10.3.11). Some transform faults connect continental parts of plates. An example is the San Andreas Fault, which extends from the southern end of the Juan de Fuca Ridge to the northern end of the East Pacific Rise (ridge) in the Gulf of California (Figures 10.28 and 10.29). The part of California west of the San Andreas Fault and all of Baja California are on the Pacific Plate. Transform faults do not just connect divergent boundaries. For example, the Queen Charlotte Fault connects the north end of the Juan de Fuca Ridge, starting at the north end of Vancouver Island, to the Aleutian subduction zone.
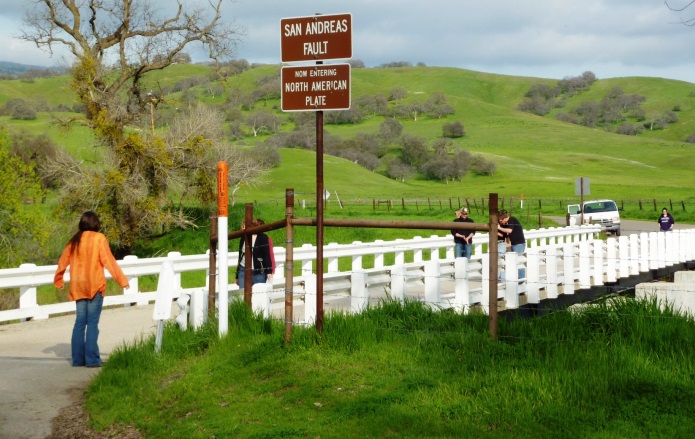
This map shows the Juan de Fuca (JDF) and Explorer Plates off the coast of Vancouver Island. We know that the JDF Plate is moving toward the North American Plate at around 4 centimeters per year to 5 centimeters per year. We think that the Explorer Plate is also moving east, but we don’t know the rate, and there is evidence that it is slower than the JDF Plate.
The boundary between the two plates is the Nootka Fault, which is the location of frequent small-to-medium earthquakes (roughly up to magnitude 5), as depicted by the red stars. Explain why the Nootka Fault is a transform fault, and show the relative sense of motion along the fault with two small arrows.
See Appendix 3 for Exercise 10.4 answers.
As originally described by Wegener in 1915, the present continents were once all part of a supercontinent, which he termed Pangea (meaning all land). More recent studies of continental matchups and the magnetic ages of ocean-floor rocks have enabled us to reconstruct the history of the break-up of Pangea.
Pangea began to rift apart along a line between Africa and Asia and between North America and South America at around 200 Ma. During the same period, the Atlantic Ocean began to open up between northern Africa and North America, and India broke away from Antarctica. Between 200 and 150 Ma, rifting started between South America and Africa and between North America and Europe, and India moved north toward Asia. By 80 Ma, Africa had separated from South America, most of Europe had separated from North America, and India had separated from Antarctica. By 50 Ma, Australia had separated from Antarctic, and shortly after that, India collided with Asia. To see the timing of these processes for yourself, go to time lapse of Continental Movements.
Within the past few million years, rifting has taken place in the Gulf of Aden and the Red Sea, and also within the Gulf of California. Incipient rifting has begun along the Great Rift Valley of eastern Africa, extending from Ethiopia and Djibouti on the Gulf of Aden (Red Sea) all the way south to Malawi.
Over the next 50 million years, it is likely that there will be full development of the east African rift and creation of new ocean floor. Eventually Africa will split apart. There will also be continued northerly movement of Australia and Indonesia. The western part of California (including Los Angeles and part of San Francisco) will split away from the rest of North America, and eventually sail right by the west coast of Vancouver Island, en route to Alaska. Because the oceanic crust formed by spreading on the mid-Atlantic ridge is not currently being subducted (except in the Caribbean), the Atlantic Ocean is slowly getting bigger, and the Pacific Ocean is getting smaller. If this continues without changing for another couple hundred million years, we will be back to where we started, with one supercontinent.
Pangea, which existed from about 350 to 200 Ma, was not the first supercontinent. It was preceded by Pannotia (600 to 540 Ma), by Rodinia (1,100 to 750 Ma), and by others before that.
In 1966, Tuzo Wilson proposed that there has been a continuous series of cycles of continental rifting and collision; that is, break-up of supercontinents, drifting, collision, and formation of other supercontinents. At present, North and South America, Europe, and Africa are moving with their respective portions of the Atlantic Ocean. The eastern margins of North and South America and the western margins of Europe and Africa are called passive margins because there is no subduction taking place along them.
This situation may not continue for too much longer, however. As the Atlantic Ocean floor gets weighed down around its margins by great thickness of continental sediments (i.e., geosynclines), it will be pushed farther and farther into the mantle, and eventually the oceanic lithosphere may break away from the continental lithosphere (Figure 10.4.12). A subduction zone will develop, and the oceanic plate will begin to descend under the continent. Once this happens, the continents will no longer continue to move apart because the spreading at the mid-Atlantic ridge will be taken up by subduction. If spreading along the mid-Atlantic ridge continues to be slower than spreading within the Pacific Ocean, the Atlantic Ocean will start to close up, and eventually (in a 100 million years or more) North and South America will collide with Europe and Africa.
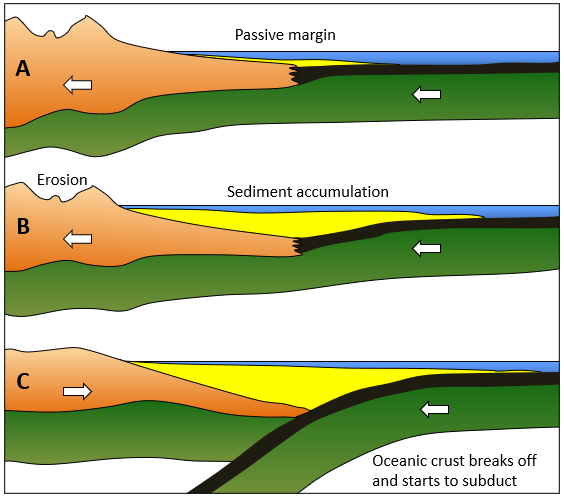
There is strong evidence around the margins of the Atlantic Ocean that this process has taken place before. The roots of ancient mountain belts, which are present along the eastern margin of North America, the western margin of Europe, and the northwestern margin of Africa, show that these land masses once collided with each other to form a mountain chain, possibly as big as the Himalayas. The apparent line of collision runs between Norway and Sweden, between Scotland and England, through Ireland, through Newfoundland, and the Maritimes, through the northeastern and eastern states, and across the northern end of Florida. When rifting of Pangea started at approximately 200 Ma, the fissuring was along a different line from the line of the earlier collision. This is why some of the mountain chains formed during the earlier collision can be traced from Europe to North America and from Europe to Africa.
That the Atlantic Ocean rift may have occurred in approximately the same place during two separate events several hundred million years apart is probably no coincidence. The series of hot spots that has been identified in the Atlantic Ocean may also have existed for several hundred million years, and thus may have contributed to rifting in roughly the same place on at least two separate occasions (Figure 10.3.13).
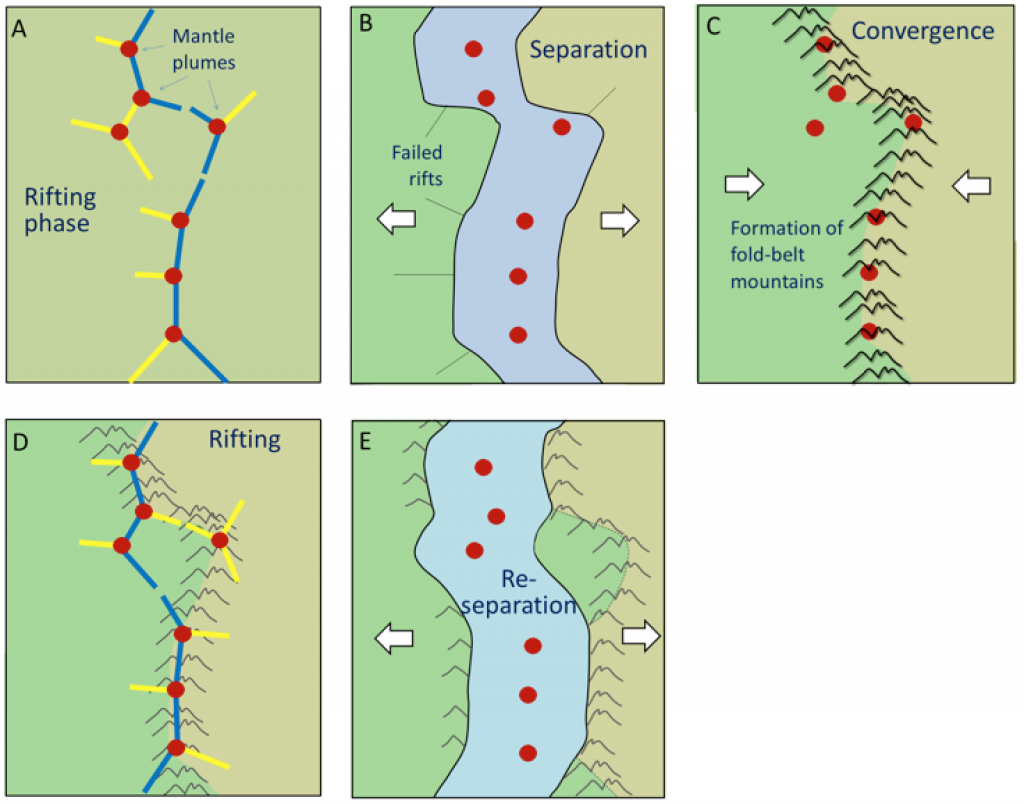
This map shows the boundaries between the major plates. Without referring to the plate map in Figure 10.4.1, or any other resources, write in the names of as many of the plates as you can. Start with the major plates, and then work on the smaller ones. Don’t worry if you can’t name them all.
Once you’ve named most of the plates, draw arrows to show the general plate motions. Finally, using a highlighter or coloured pencil, label as many of the boundaries as you can as divergent, convergent, or transform.
See Appendix 3 for Exercise 10.5 answers.
Image descriptions
Media Attributions
- Figure 10.4.1: “Plates tect2 en” by the USGS. Adapted by Steven Earle. Public domain.
- Figures 10.4.2, 10.4.3, 10.4.5, 10.4.6, 10.4.7, 10.4.8, 10.4.9, 10.4.10, 10.4.11, 10.4.12, 10.4.13, 10.4.14: © Steven Earle. CC BY.
- Figure 10.4.4: © Steven Earle. CC BY. Based on Keary and Vine, 1996, Global Tectonics (2ed), Blackwell Science Ltd., Oxford.